Chen Tao, Ningbo Institute of Materials,
QQ Academic Group: 1092348845
Detailed
Aggregation-induced emission (AIE) active fluorescent polymer hydrogel (FPH) is a combination of AIE active material and polymer hydrogel. Unlike the extensively studied AIE active materials, which are mainly used in solution or dry solid state, they have a three-dimensional cross-linked polymer network that can absorb water without dissolving. Therefore, they are known to have many favorable properties, such as soft wetness, tissue-like mechanical strength, biocompatibility, biomimetic self-repair function, easy-to-customize structure, and responsive fluorescence and volume/shape changes, so they represent A promising category of luminescent materials with many cutting-edge uses. Recently, researcher Chen Tao from Ningbo Institute of Materials Technology and Engineering, Chinese Academy of Sciences reviewed an article titled Progress in aggregation-induced emission-active fluorescent polymeric hydrogels in Aggregate. This review aims to systematically summarize the latest developments in this young but thriving field of research, with particular emphasis on its design and preparation. The current challenges and future prospects in the field were also discussed to attract new interest and stimulate more efforts.
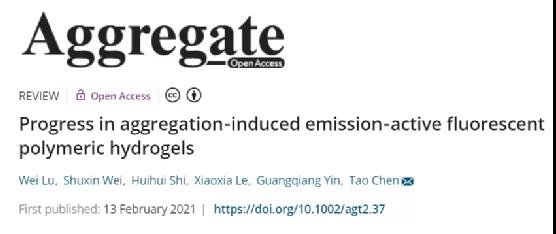
Introduction: In nature, a large number of shells and tissues of marine mollusks are found to exist in the state of quasi-solid hydrogel, and they have evolved to use intelligent light-emitting behavior to communicate, camouflage or reproduce. When there is a threat, the bioluminescence intensity will change significantly. Inspired by these interesting natural systems, people have made great efforts to introduce fluorescent parts into polymer hydrogels to produce fluorescent polymer hydrogels (FPH) with adjustable fluorescence color/intensity characteristics .
So far, although the research on AIE-active FPH is still in its infancy, there have been many elegant research reports. However, there are currently no such reviews summarizing the progress of these flourishing materials. In this article, the author attempts to systematically summarize the structure and fluorescence properties of AIE-active FPH. Since most of the reported FPH is based on organic AIEgen, FPH is mainly classified according to synthesis strategy, including physical doping, covalent bonding, and supramolecular polymerization cross-linked polymer network of organic AIEgen (schematic diagram 1). Subsequently, some important examples of the preparation of AIE active inorganic-organic hybrid metal nanoclusters are briefly introduced. It also discussed current challenges and future perspectives.
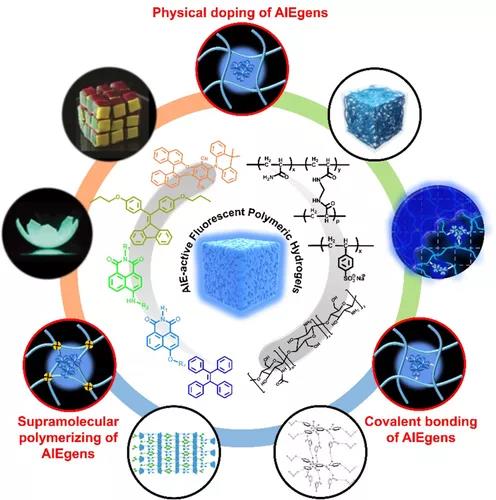
Diagram 1 shows the synthesis strategy of aggregation-induced emission (AIE) active fluorescent polymer hydrogel (FPH)
Based on the agglomeration-induced emissive polymer hydrates of organics, AIEgens are physically doped into polymer hydrogels: Generally, most of the reported organic AIEgens are hydrophobic, so it is easy to form fluorescent agglomerates. The emission is greatly enhanced in an aqueous matrix. Therefore, they are ideal building blocks for constructing highly fluorescent polymer hydrogels. To this end, the most straightforward strategy is to blend an AIE molecule directly into the crosslinked polymer network. For example, Wang and colleagues proposed a series of propeller-shaped bis(4-propoxyphenyl)-dibenzofullerenes (DBF) physically incorporated into chemically crosslinked polyacrylamide hydrogels. Smart luminescent hydrogel that responds to fluorescent color changes (Figure 1A). Since acetonitrile is a good solvent for DBF, the introduction of acetonitrile into the hydrogel matrix will greatly affect the degree of aggregation of organic AIEgens. As a result, according to the volume ratio of water/acetonitrile, the emission color of the prepared hydrogel can be easily adjusted to cover orange, yellow, green and blue (Figure 1B, C).
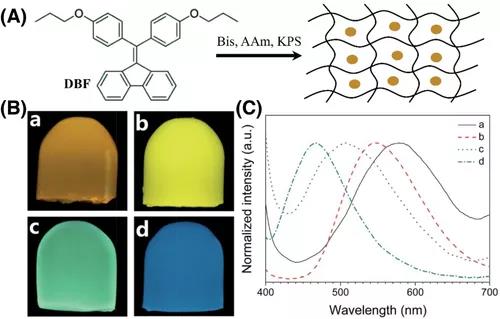
Figure 1 (A) The chemical structure of DBF and the schematic diagram of the hydrogel. Bis, AAm and KPS are bisacrylamide, acrylamide and potassium persulfate respectively. (B) Photographs of hydrogel samples with different water content (a: 90%, b: 70%, c: 60%, d: after freezing in liquid N2 and melting at room temperature), and (C) respective Fluorescence Spectroscopy.
In addition, Ji and his colleagues physically incorporated several different colors of AIEgens into a cross-linked polymer hydrogel prepared from acylhydrazine-terminated polyethylene oxide (PEO) and tetraaldehyde-terminated PEO (Figure 2A, B). Due to the dynamic and reversible nature of the formed acyl hydr bond, the obtained multi-color fluorescent hydrogel film can be easily combined with a non-fluorescent hydrogel cube to form a functionally macroscopic "Rubic Cube (RC)" "(Figure 2C). Interestingly, the behavior of this hydrogel analog is similar to real RC, where the attached fluorescent hydrogel film can be easily rotated by hand to produce interchangeable multi-color fluorescent patterns (Figure 2D), Use can be found in selective colorimetric sensor arrays and information storage. By using similar AIEgen, they have further created muscle-like structured hydrogels that exhibit unprecedented selective self-repair behavior guided by AIEgen.
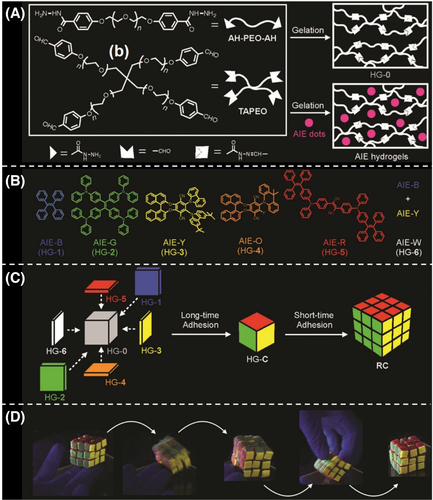
Figure 2 (A) This figure shows the preparation of fluorescent polymer hydrogels of different colors by physically doping various organic AIEgens into acyl-hydr-bonded PEO hydrogels. (B) The chemical structure of these organic AIEgens. (C) Explain the scheme of Rubiks cube-shaped FPH structure. (D) The image shows the process of generating a variable pattern by rotating the Rubiks Cube FPH horizontally and vertically.
Recently, they incorporated pH-responsive AIEgen (tetra(4-pyridylphenyl)ethylene, TPE-4Py) into a cross-linked poly(acrylamide/sodium 4-styrene sulfonate) network to develop biomimetic fluorescence Hydrogel actuator (Figure 3A). Under acidic conditions, the TPE-4Py molecule is protonated, so it can stably bind to the negatively charged benzenesulfonate group on the polymer chain (Figure 3B). When the environment becomes neutral or alkaline, hydrophobic TPE-4Py tends to form aggregates, resulting in emission color changes and volume shrinkage of the active layer. As a result, for a specially designed three-arm double-layer hydrogel actuator, pH-triggered coordinated emission color/shape changes were observed (Figure 3C).
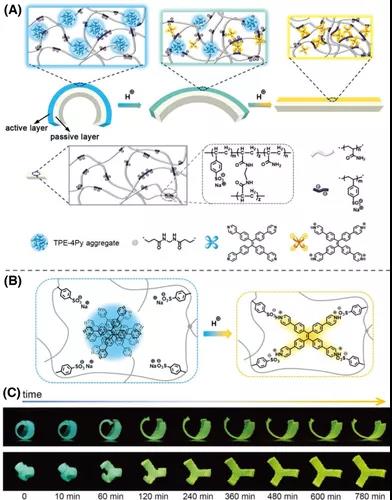
Figure 3(A) shows the scheme of the cooperative shape and fluorescence color change of the double-layer hydrogel actuator based on TPE-4Py/PAS. The chemical structures of the hydrogel and TPE-4Py AIEgen are also given. (B) The proposed mechanism of pH-sensitive fluorescence color change. (C) The three-arm double-layer actuator simultaneously undergoes fluorescent color changes and complex shape deformations.
Covalent bonding of AIEgens to polymer hydrogels: Hydrophobic organic AIEgens have also been covalently grafted onto crosslinked polymer chains to produce FPH. This covalent bonding strategy helps avoid accidental leakage of small molecule AIEgens, which may be encountered by FPH prepared by the above-mentioned physical incorporation strategy. In previous contributions, the author used N,N-dimethylethylenediamine instead of naphthalimide (NI) luminescent agent to obtain 4-(N,N-dimethylaminoethylene) amino group with AIE activity -N-allyl-1,8-naphthalimide (DEAN) monomer has a propeller-like configuration. Further research shows that the fluorescence intensity of DEAN can also be controlled by Zn2+ and Al3+, but in different ways (Figure 4).
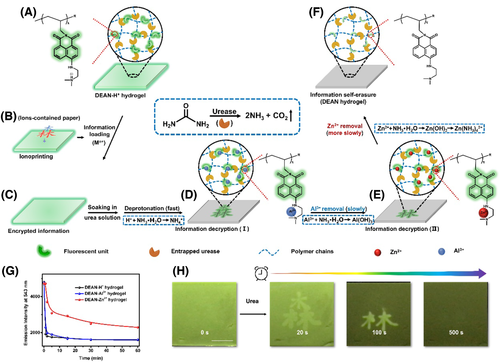
Figure 4 (A) Schematic diagram showing the preparation of a naphthalimide photoluminescence FPH system for instant information storage. (B) The process of encrypting information by partially ionizing the printed hydrogel with Zn2+ and Al3+ that can chelate the naphthalimide part. (CF) The gradual decryption and self-erasing process of the encoded information in the urea solution generates NH3 through an enzymatic reaction, which can decompose the complex. (G) The relationship between the change of peak fluorescence intensity of DEAN-H+, DEAN-Zn2+ and DEAN-Al3+ hydrogels at 543 nm and the immersion time in urea solution. (H) A photo showing the progressive information decryption and self-erasing.
Even if AIEgens itself is not sensitive to external stimuli, it is still possible to obtain FPH with tunable fluorescence behavior, because the degree of aggregation of AIEgens can be easily adjusted by the conformational changes of the polymer chains in response to some external stimuli. Recently, a typical system was proposed by copolymerizing phenoxy-substituted naphthalimide monomers (PhAN) into a thermally responsive PNIPAM network. It has weak fluorescence at room temperature, but due to the shrinkage of the PNIPAM polymer network grafted with naphthalimide and the subsequent more compact NI aggregation, it emits a strong intensity above the volume phase transition temperature (about 33°C) Blu-ray. However, this FPH example is limited to two-state switching of fluorescence characteristics (switch emission response when temperature changes), thereby limiting its potential use in on-demand information display or anti-counterfeiting. For this reason, another interesting dual-network FPH example was further developed. As shown in Figure 5A, the system is based on the free radical polymerization of dimethylaminoethoxy-substituted naphthalimide monomer (DAEAN) to chemically crosslinked poly(acrylic acid-co-acrylamide) (poly (AAc-AAm)) formed in the network, the network is hydrogen-bonded agar network.
The inset of Figure 5(A) shows the chemical structure of PAAD hydrogel grafted with AIE-active naphthalimide luminophore as the side group, and the proposed thermal response polymorphic fluorescence reaction mechanism. (B) FPH-based on-demand decryption 3D anti-counterfeiting platform, which is prepared by folding a 2D information carrier into a 3D shape by hand, and then immersing it in Eu3+ solution to fix this 3D hydrogel structure through a shape memory process. Different messages will appear at 40°C ("wood") and 70°C ("village"). When ethylenediaminetetraacetic acid disodium salt is used to break the Eu3+-AAc crosslink to induce the shape to return from 3D to 2D, the entire message ("tree") is decrypted.
Since the conformational changes of the crosslinked polymer chains can affect the fluorescence properties of grafted AIEgens, people began to wonder whether the highly sensitive AIEgens fluorescence color/intensity changes can be used to visually track the gelation process of the polymer. As detailed in the exquisite work reported by Wang et al., the answer is yes. As shown in Figure 6A, they labeled chitosan with TPE luminescent element as a side group, which is referred to as TPE-CS. For the TPE-CS solution, no specific fluorescence pattern was found in the confocal laser scanning fluorescence microscope image (Figure 6C). As the thermal gelation progressed, some bright areas gradually appeared and continued to grow over time (Figure 6D–F).
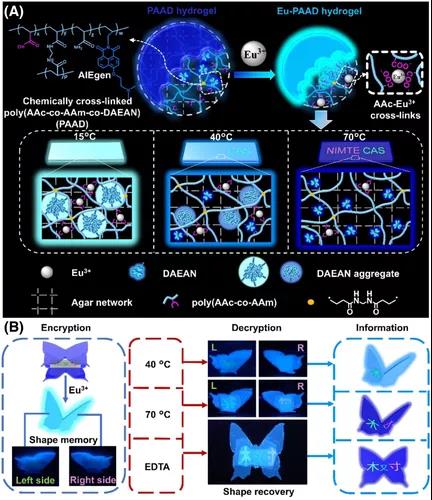
Figure 6 (A) The chemical structure of TPE-functionalized chitosan polymer TPE-CS. (B) The XRD pattern recorded during the gelation of TPE-CS illustrates the evolution of the crystal. (CF) Confocal laser scanning fluorescence microscope image recorded during the gelation process from solution to hydrogel. The scale of (CE) is 250μm, and the scale of (F) is 25μm. The diagram (G–J) shows the formation of crosslinks during the gel process.
Another general method for producing AIE-active FPH is to covalently attach organic AIEgen to the end of a cross-linked polymer chain. Zhang et al. reported an impressive example. This material is obtained by combining a salt-responsive peptide with a TPE luminescent agent. In the presence of NaCl, the monodisperse peptide oligomers will self-assemble into a bright blue polymer hydrogel. However, the formation of this salt-responsive peptide hydrogel is limited to a one-way process and is irreversible. Therefore, they redesigned the system by changing the peptide sequence to the TPE-MAX shown in Figure 7A. Due to its pH-responsive properties, the replacement peptide can self-assemble into a cross-linked polymer structure with strong fluorescence through intermolecular association of non-polar residues in alkaline solution (pH 10.0) (Figure 7B), resulting in TPE Part of the mass gathering. When the pH is adjusted to acidic conditions (pH 6.0), the blue-emitting hydrogel recombines into the weakly emitting solution (Figure 7C, D).
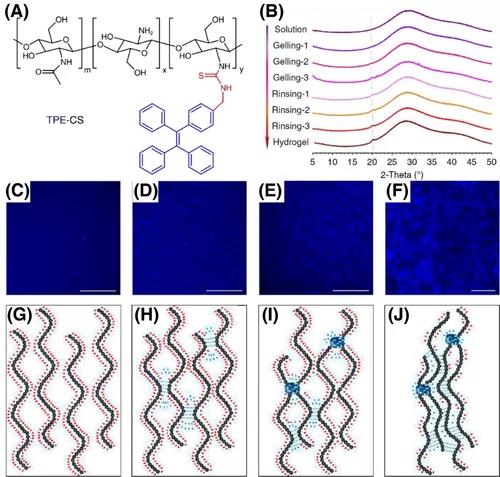
Figure 7 (A) The chemical structure of TPE terminal peptide TPE-MAX. (B) The proposed mechanism of its pH-sensitive gelling process. (C) A photograph showing the pH-sensitive sol-gel transition process, and (D) the fluorescence spectra of the hydrogel and solution.
AIE-active FPH through supramolecular polymerization: AIE-active FPH can also be constructed from low molecular weight AIEgen-containing monomer units and comonomer molecules through the spontaneous self-assembly process of supramolecular polymerization. For example, Wang et al. proposed a supermolecular cross-linking based on electrostatic interaction between polysodium p-styrene sulfonate (1) and a tetraphenylethylene derivative functionalized with two quaternary ammonium groups (2). Responsive supramolecular fluorescent hydrogel (Figure 9A).
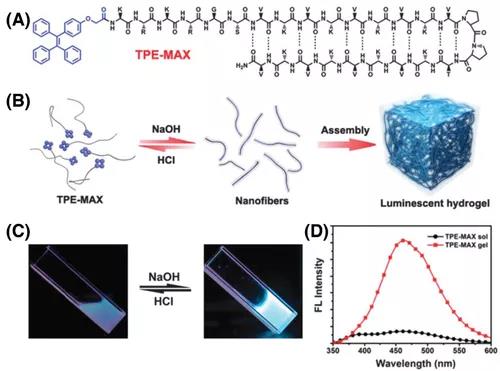
Figure 9 (A) is a schematic diagram showing the gel-sol transition process of preparing AIE-active FPH through electrostatic interaction and its bioresponsiveness. (B) Cytotoxicity of 1, 2 and Hydrogel 3, which were determined by standard MTT analysis using HEK293 cell line. (C) Confocal laser scanning microscope image of HEK293 cells incubated with hydrogel 3 (scale bar: 20 μm).
Host-guest interaction is another important driving force for supramolecular polymerization and crosslinking. Due to its dynamic and reversible characteristics, AIE active supramolecular polymer hydrogels based on host-guest interaction usually not only respond to a variety of external stimuli, but also have satisfactory self-repair properties, which are crucial for long-term service important. Li and his colleagues recently reported an impressive example (Figure 10). The example is that bis-(β-cyclodextrin) (β-CD) bridged by TPE and pyridine-containing adamantane molecules interact with the host. The interaction between the guests and the subsequent Zn2+-pyridine-pyridine coordination interaction.
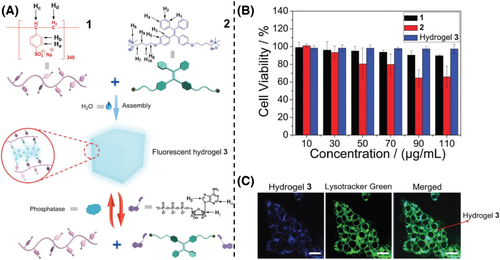
Figure 10 (A) is a schematic diagram showing the production of AIE active FPH through Host-Guest interaction, as well as a cartoon showing a protected quick response (QR) code, which has enhanced durability and security.
Active AIE polymer hydrates prepared by inorganic-organic hybrid metal nanoclusters: Although the AIE phenomenon was first discovered in organic molecules with a propeller-like conformation, it has recently been extended to luminescent metal nanoclusters (NCs). Metal cations are reduced in the presence of various ligands or through metal cations and ligands. As shown in Figure 13, the water-soluble coordinated Ag NCs were prepared in the alkaline aqueous solution of AgNO3 and thiosalicylic acid. After adding an anti-solvent (such as ethanol), the aqueous solution of Ag NCs tends to self-assemble into highly ordered fibers, and at the same time gelatinize to form a cross-linked network, and enhance the aggregated photoluminescence.
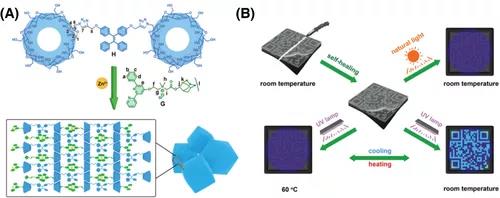
Figure 13 (A) The synthesis procedure of Ag NCs, the ESI-MS characterization and the magnification of the experimental and simulated isotope distributions of the designated peaks. (B) After adding EtOH, the change of self-assembly with time and a schematic diagram showing the gelation process. (C) The optical properties of the gel with the addition of EtOH and the emission curve recorded at different times.
Paper link:OI.org/10.1002/Argentina 2.37
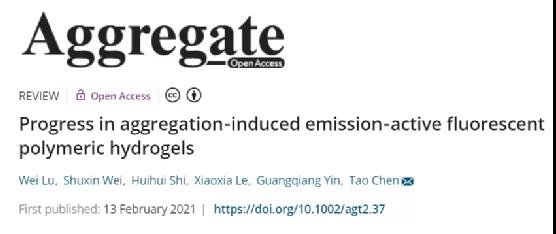
Introduction: In nature, a large number of shells and tissues of marine mollusks are found to exist in the state of quasi-solid hydrogel, and they have evolved to use intelligent light-emitting behavior to communicate, camouflage or reproduce. When there is a threat, the bioluminescence intensity will change significantly. Inspired by these interesting natural systems, people have made great efforts to introduce fluorescent parts into polymer hydrogels to produce fluorescent polymer hydrogels (FPH) with adjustable fluorescence color/intensity characteristics .
So far, although the research on AIE-active FPH is still in its infancy, there have been many elegant research reports. However, there are currently no such reviews summarizing the progress of these flourishing materials. In this article, the author attempts to systematically summarize the structure and fluorescence properties of AIE-active FPH. Since most of the reported FPH is based on organic AIEgen, FPH is mainly classified according to synthesis strategy, including physical doping, covalent bonding, and supramolecular polymerization cross-linked polymer network of organic AIEgen (schematic diagram 1). Subsequently, some important examples of the preparation of AIE active inorganic-organic hybrid metal nanoclusters are briefly introduced. It also discussed current challenges and future perspectives.
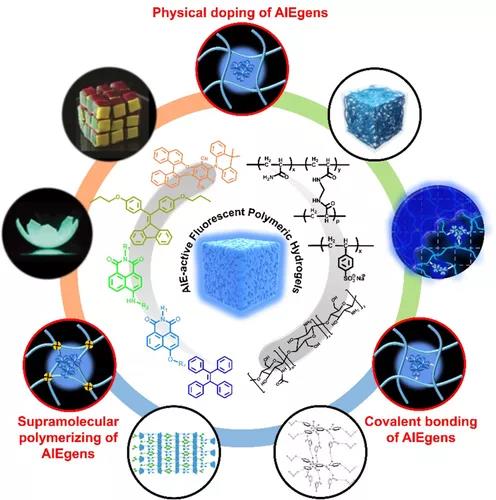
Diagram 1 shows the synthesis strategy of aggregation-induced emission (AIE) active fluorescent polymer hydrogel (FPH)
Based on the agglomeration-induced emissive polymer hydrates of organics, AIEgens are physically doped into polymer hydrogels: Generally, most of the reported organic AIEgens are hydrophobic, so it is easy to form fluorescent agglomerates. The emission is greatly enhanced in an aqueous matrix. Therefore, they are ideal building blocks for constructing highly fluorescent polymer hydrogels. To this end, the most straightforward strategy is to blend an AIE molecule directly into the crosslinked polymer network. For example, Wang and colleagues proposed a series of propeller-shaped bis(4-propoxyphenyl)-dibenzofullerenes (DBF) physically incorporated into chemically crosslinked polyacrylamide hydrogels. Smart luminescent hydrogel that responds to fluorescent color changes (Figure 1A). Since acetonitrile is a good solvent for DBF, the introduction of acetonitrile into the hydrogel matrix will greatly affect the degree of aggregation of organic AIEgens. As a result, according to the volume ratio of water/acetonitrile, the emission color of the prepared hydrogel can be easily adjusted to cover orange, yellow, green and blue (Figure 1B, C).
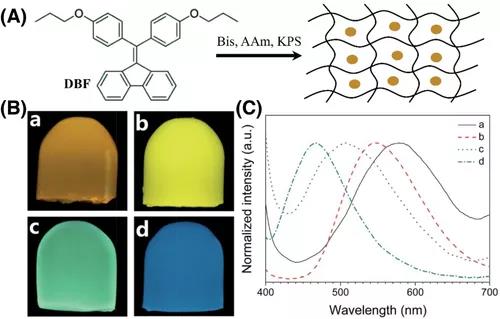
Figure 1 (A) The chemical structure of DBF and the schematic diagram of the hydrogel. Bis, AAm and KPS are bisacrylamide, acrylamide and potassium persulfate respectively. (B) Photographs of hydrogel samples with different water content (a: 90%, b: 70%, c: 60%, d: after freezing in liquid N2 and melting at room temperature), and (C) respective Fluorescence Spectroscopy.
In addition, Ji and his colleagues physically incorporated several different colors of AIEgens into a cross-linked polymer hydrogel prepared from acylhydrazine-terminated polyethylene oxide (PEO) and tetraaldehyde-terminated PEO (Figure 2A, B). Due to the dynamic and reversible nature of the formed acyl hydr bond, the obtained multi-color fluorescent hydrogel film can be easily combined with a non-fluorescent hydrogel cube to form a functionally macroscopic "Rubic Cube (RC)" "(Figure 2C). Interestingly, the behavior of this hydrogel analog is similar to real RC, where the attached fluorescent hydrogel film can be easily rotated by hand to produce interchangeable multi-color fluorescent patterns (Figure 2D), Use can be found in selective colorimetric sensor arrays and information storage. By using similar AIEgen, they have further created muscle-like structured hydrogels that exhibit unprecedented selective self-repair behavior guided by AIEgen.
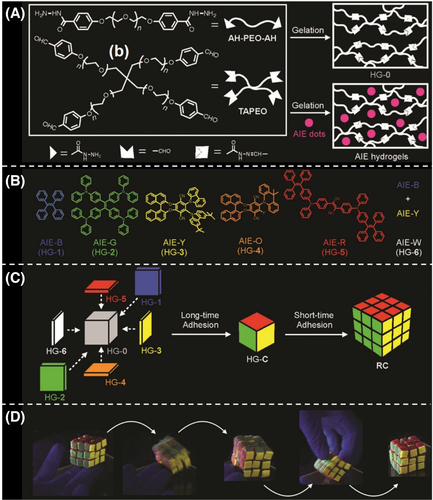
Figure 2 (A) This figure shows the preparation of fluorescent polymer hydrogels of different colors by physically doping various organic AIEgens into acyl-hydr-bonded PEO hydrogels. (B) The chemical structure of these organic AIEgens. (C) Explain the scheme of Rubiks cube-shaped FPH structure. (D) The image shows the process of generating a variable pattern by rotating the Rubiks Cube FPH horizontally and vertically.
Recently, they incorporated pH-responsive AIEgen (tetra(4-pyridylphenyl)ethylene, TPE-4Py) into a cross-linked poly(acrylamide/sodium 4-styrene sulfonate) network to develop biomimetic fluorescence Hydrogel actuator (Figure 3A). Under acidic conditions, the TPE-4Py molecule is protonated, so it can stably bind to the negatively charged benzenesulfonate group on the polymer chain (Figure 3B). When the environment becomes neutral or alkaline, hydrophobic TPE-4Py tends to form aggregates, resulting in emission color changes and volume shrinkage of the active layer. As a result, for a specially designed three-arm double-layer hydrogel actuator, pH-triggered coordinated emission color/shape changes were observed (Figure 3C).
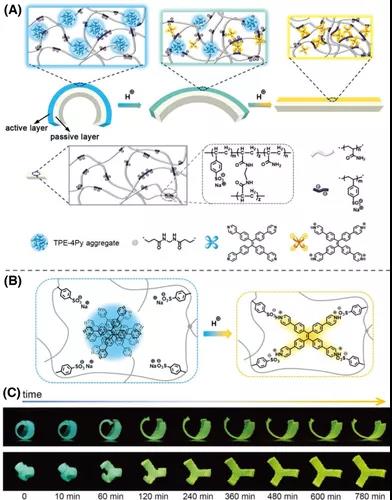
Figure 3(A) shows the scheme of the cooperative shape and fluorescence color change of the double-layer hydrogel actuator based on TPE-4Py/PAS. The chemical structures of the hydrogel and TPE-4Py AIEgen are also given. (B) The proposed mechanism of pH-sensitive fluorescence color change. (C) The three-arm double-layer actuator simultaneously undergoes fluorescent color changes and complex shape deformations.
Covalent bonding of AIEgens to polymer hydrogels: Hydrophobic organic AIEgens have also been covalently grafted onto crosslinked polymer chains to produce FPH. This covalent bonding strategy helps avoid accidental leakage of small molecule AIEgens, which may be encountered by FPH prepared by the above-mentioned physical incorporation strategy. In previous contributions, the author used N,N-dimethylethylenediamine instead of naphthalimide (NI) luminescent agent to obtain 4-(N,N-dimethylaminoethylene) amino group with AIE activity -N-allyl-1,8-naphthalimide (DEAN) monomer has a propeller-like configuration. Further research shows that the fluorescence intensity of DEAN can also be controlled by Zn2+ and Al3+, but in different ways (Figure 4).
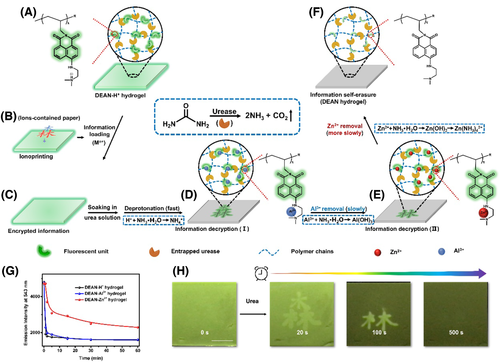
Figure 4 (A) Schematic diagram showing the preparation of a naphthalimide photoluminescence FPH system for instant information storage. (B) The process of encrypting information by partially ionizing the printed hydrogel with Zn2+ and Al3+ that can chelate the naphthalimide part. (CF) The gradual decryption and self-erasing process of the encoded information in the urea solution generates NH3 through an enzymatic reaction, which can decompose the complex. (G) The relationship between the change of peak fluorescence intensity of DEAN-H+, DEAN-Zn2+ and DEAN-Al3+ hydrogels at 543 nm and the immersion time in urea solution. (H) A photo showing the progressive information decryption and self-erasing.
Even if AIEgens itself is not sensitive to external stimuli, it is still possible to obtain FPH with tunable fluorescence behavior, because the degree of aggregation of AIEgens can be easily adjusted by the conformational changes of the polymer chains in response to some external stimuli. Recently, a typical system was proposed by copolymerizing phenoxy-substituted naphthalimide monomers (PhAN) into a thermally responsive PNIPAM network. It has weak fluorescence at room temperature, but due to the shrinkage of the PNIPAM polymer network grafted with naphthalimide and the subsequent more compact NI aggregation, it emits a strong intensity above the volume phase transition temperature (about 33°C) Blu-ray. However, this FPH example is limited to two-state switching of fluorescence characteristics (switch emission response when temperature changes), thereby limiting its potential use in on-demand information display or anti-counterfeiting. For this reason, another interesting dual-network FPH example was further developed. As shown in Figure 5A, the system is based on the free radical polymerization of dimethylaminoethoxy-substituted naphthalimide monomer (DAEAN) to chemically crosslinked poly(acrylic acid-co-acrylamide) (poly (AAc-AAm)) formed in the network, the network is hydrogen-bonded agar network.
The inset of Figure 5(A) shows the chemical structure of PAAD hydrogel grafted with AIE-active naphthalimide luminophore as the side group, and the proposed thermal response polymorphic fluorescence reaction mechanism. (B) FPH-based on-demand decryption 3D anti-counterfeiting platform, which is prepared by folding a 2D information carrier into a 3D shape by hand, and then immersing it in Eu3+ solution to fix this 3D hydrogel structure through a shape memory process. Different messages will appear at 40°C ("wood") and 70°C ("village"). When ethylenediaminetetraacetic acid disodium salt is used to break the Eu3+-AAc crosslink to induce the shape to return from 3D to 2D, the entire message ("tree") is decrypted.
Since the conformational changes of the crosslinked polymer chains can affect the fluorescence properties of grafted AIEgens, people began to wonder whether the highly sensitive AIEgens fluorescence color/intensity changes can be used to visually track the gelation process of the polymer. As detailed in the exquisite work reported by Wang et al., the answer is yes. As shown in Figure 6A, they labeled chitosan with TPE luminescent element as a side group, which is referred to as TPE-CS. For the TPE-CS solution, no specific fluorescence pattern was found in the confocal laser scanning fluorescence microscope image (Figure 6C). As the thermal gelation progressed, some bright areas gradually appeared and continued to grow over time (Figure 6D–F).
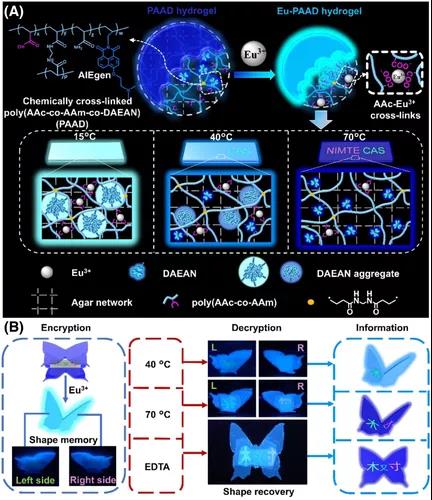
Figure 6 (A) The chemical structure of TPE-functionalized chitosan polymer TPE-CS. (B) The XRD pattern recorded during the gelation of TPE-CS illustrates the evolution of the crystal. (CF) Confocal laser scanning fluorescence microscope image recorded during the gelation process from solution to hydrogel. The scale of (CE) is 250μm, and the scale of (F) is 25μm. The diagram (G–J) shows the formation of crosslinks during the gel process.
Another general method for producing AIE-active FPH is to covalently attach organic AIEgen to the end of a cross-linked polymer chain. Zhang et al. reported an impressive example. This material is obtained by combining a salt-responsive peptide with a TPE luminescent agent. In the presence of NaCl, the monodisperse peptide oligomers will self-assemble into a bright blue polymer hydrogel. However, the formation of this salt-responsive peptide hydrogel is limited to a one-way process and is irreversible. Therefore, they redesigned the system by changing the peptide sequence to the TPE-MAX shown in Figure 7A. Due to its pH-responsive properties, the replacement peptide can self-assemble into a cross-linked polymer structure with strong fluorescence through intermolecular association of non-polar residues in alkaline solution (pH 10.0) (Figure 7B), resulting in TPE Part of the mass gathering. When the pH is adjusted to acidic conditions (pH 6.0), the blue-emitting hydrogel recombines into the weakly emitting solution (Figure 7C, D).
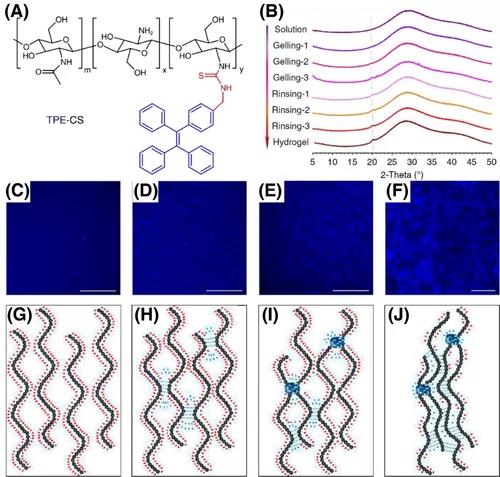
Figure 7 (A) The chemical structure of TPE terminal peptide TPE-MAX. (B) The proposed mechanism of its pH-sensitive gelling process. (C) A photograph showing the pH-sensitive sol-gel transition process, and (D) the fluorescence spectra of the hydrogel and solution.
AIE-active FPH through supramolecular polymerization: AIE-active FPH can also be constructed from low molecular weight AIEgen-containing monomer units and comonomer molecules through the spontaneous self-assembly process of supramolecular polymerization. For example, Wang et al. proposed a supermolecular cross-linking based on electrostatic interaction between polysodium p-styrene sulfonate (1) and a tetraphenylethylene derivative functionalized with two quaternary ammonium groups (2). Responsive supramolecular fluorescent hydrogel (Figure 9A).
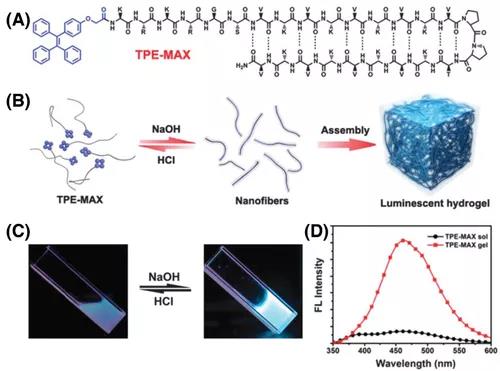
Figure 9 (A) is a schematic diagram showing the gel-sol transition process of preparing AIE-active FPH through electrostatic interaction and its bioresponsiveness. (B) Cytotoxicity of 1, 2 and Hydrogel 3, which were determined by standard MTT analysis using HEK293 cell line. (C) Confocal laser scanning microscope image of HEK293 cells incubated with hydrogel 3 (scale bar: 20 μm).
Host-guest interaction is another important driving force for supramolecular polymerization and crosslinking. Due to its dynamic and reversible characteristics, AIE active supramolecular polymer hydrogels based on host-guest interaction usually not only respond to a variety of external stimuli, but also have satisfactory self-repair properties, which are crucial for long-term service important. Li and his colleagues recently reported an impressive example (Figure 10). The example is that bis-(β-cyclodextrin) (β-CD) bridged by TPE and pyridine-containing adamantane molecules interact with the host. The interaction between the guests and the subsequent Zn2+-pyridine-pyridine coordination interaction.
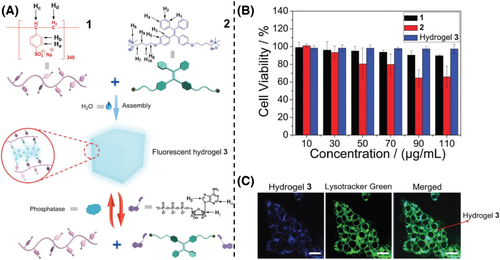
Figure 10 (A) is a schematic diagram showing the production of AIE active FPH through Host-Guest interaction, as well as a cartoon showing a protected quick response (QR) code, which has enhanced durability and security.
Active AIE polymer hydrates prepared by inorganic-organic hybrid metal nanoclusters: Although the AIE phenomenon was first discovered in organic molecules with a propeller-like conformation, it has recently been extended to luminescent metal nanoclusters (NCs). Metal cations are reduced in the presence of various ligands or through metal cations and ligands. As shown in Figure 13, the water-soluble coordinated Ag NCs were prepared in the alkaline aqueous solution of AgNO3 and thiosalicylic acid. After adding an anti-solvent (such as ethanol), the aqueous solution of Ag NCs tends to self-assemble into highly ordered fibers, and at the same time gelatinize to form a cross-linked network, and enhance the aggregated photoluminescence.
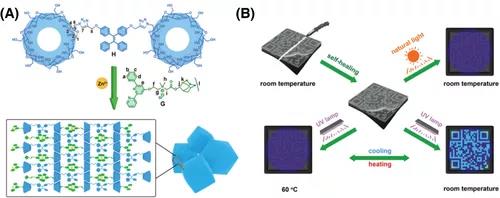
Figure 13 (A) The synthesis procedure of Ag NCs, the ESI-MS characterization and the magnification of the experimental and simulated isotope distributions of the designated peaks. (B) After adding EtOH, the change of self-assembly with time and a schematic diagram showing the gelation process. (C) The optical properties of the gel with the addition of EtOH and the emission curve recorded at different times.
Paper link:OI.org/10.1002/Argentina 2.37
- Previous: AM: Low-temperature de
- Next: A Rising 2D Star: Nove