[Hot article review] Research progress and prospects of battery energy storage technology
QQ Academic Group: 1092348845
Detailed
Keywords: battery energy storage; lithium ion battery; flow battery; sodium-sulfur battery; evaluation standard; hybrid energy storage
Energy storage refers to a technology that stores energy in the form of electricity, etc., in a certain form through different media, and releases it for work (generation, etc.) when needed. Energy storage technology is one of the core technologies to promote clean, electrified and efficient world energy, break energy resources and environmental constraints, and achieve global energy transformation and upgrading. Facing the future high penetration of new energy access and consumption, it is necessary to build a high-proportion, ubiquitous, and wide-area collaborative energy storage form, and to change the form, structure and function of the traditional power system through new energy plus energy storage. To promote the sustainable development of clean energy in a solid and orderly manner, it is necessary to rely on energy storage technology with low border costs. As shown in Figure 1, energy storage technologies are divided into three categories: physical energy storage, electrochemical energy storage (battery energy storage) and chemical energy storage (such as hydrogen, hydrocarbon, and hydrocarbon energy storage). Physical energy storage mainly includes pumped water storage, compressed air energy storage, flywheel energy storage, and heat storage. Among them, pumped storage technology is relatively mature, and the cumulative installed capacity of energy storage projects that have been put into operation globally and domestically accounts for more than 93%. However, the technology is limited by geographical conditions, which affects its wide application. Hydrogen has a wide range of sources, large reserves, pollution-free, and high energy density. It is widely regarded as the most promising secondary energy source in the future. Hydrogen energy storage technology is a kind of use of hydrogen as an energy storage medium, and redundancy is used when electricity is overproduction. Electricity produces hydrogen and stores it. When the grid power is insufficient, the stored hydrogen is used to produce electricity through fuel cells or converted into methane to power conventional gas turbine generators. Domestic and foreign countries attach great importance to hydrogen storage technology, but there is still a long way to go in terms of key technology, material research and development, and system integration.
Compared with physical energy storage and chemical energy storage, battery energy storage has more advantages in terms of scalability, service life, and flexibility. Battery energy storage is mainly based on energy storage technologies such as lithium-ion batteries, flow batteries, lead-acid batteries and sodium-based batteries, as shown in Figure 2(a). According to the China Energy Storage Alliance (CNESA) ) According to incomplete statistics of the global energy storage project library, as of the end of 2018, the global cumulative installed capacity of lithium-ion batteries accounted for 82%, followed by sodium-based batteries, lead storage batteries and flow batteries. The cumulative installed power scale of the global electrochemical energy storage market is 6058.9 MW, as shown in Figure 2(b), with a compound growth rate of 62% in 2019, continuing to maintain a rapid growth trend. Currently, lithium-ion batteries are the most successful portable energy storage batteries, but their use is limited to small electronic devices. In large-scale energy storage, lithium-ion batteries are limited by performance, cost, and safety.
In view of the problems in the development of battery energy storage in the early stage and the future development needs of battery energy storage technology, this article first puts forward the evaluation criteria of energy storage technology, reviews the history of battery energy storage body development, and summarizes the existing battery energy storage Technology and forecast the future development direction of energy storage technology.
1 Evaluation criteria for energy storage technology
Currently, there is no energy storage technology that can meet all the needs of new energy grids. Due to different working principles and different application scenarios, the advantages and limitations of each energy storage technology are also different. On January 16, 2020, the General Office of the National Energy Administration, the General Office of the Emergency Management Department, and the General Office of the State Administration for Market Regulation jointly formulated the "Implementation Plan on Strengthening Energy Storage Standardization" to further promote the implementation of the "Regarding the Promotion of Energy Storage Technology "Guiding Opinions on Industrial Development" (Fagai Energy [2017] No. 1701), strengthen the standardization of energy storage, play the role of standardization and leadership, and promote the high-quality development of the energy storage industry. For industry development, standards first. Related associations have set out to formulate some industry standards for energy storage, but the industry’s evaluation standards for energy storage technology are still relatively vague, and it is necessary to sort them out and put forward common evaluation standards that meet objective and actual needs.
As early as 2008, Ibrahim et al. compared the existing energy storage technologies in terms of technical indicators (power, energy efficiency, volume, etc.) and economy (investment cost), and proposed correspondingly suitable energy storage technologies based on application scenarios. Energy storage technology has formed a preliminary evaluation index for energy storage. In 2011, Yu Enke and others analyzed various energy storage technologies mainly through the comparison of technical indicators, and pointed out that emerging chemical energy storage such as flow batteries and sodium-sulfur batteries were the most suitable electrochemical energy storage technologies for large-scale development at that time. In 2011, Fang Tong and others from the State Grid Energy Research Institute established a comprehensive evaluation index system for battery energy storage technology through three aspects: technology, economy and environment, and used the analytic hierarchy process. In addition, Makarov et al., Liu Shinian et al., Yan Junchen et al. also discussed the evaluation of energy storage technology.
In recent years, energy storage technology has been continuously updated and iterated, and application scenarios have been continuously enriched. On the basis of combining the needs of large-scale energy storage technology and previous studies, this article summarizes four evaluation criteria for evaluating and comparing energy storage technologies and products, as shown in Figure 3.
Figure 1 Power and discharge rate of each energy storage technology
Figure 2 (a) Proportion of cumulative installed capacity of various battery energy storage technologies worldwide and (b) cumulative installed capacity of electrochemical energy storage in recent years
Figure 3 Evaluation criteria for energy storage technology
(1) Safety: During the entire life cycle, the energy storage system remains in good condition and does not pose a threat to the human body under normal conditions of use and accidents. Safety is the first and basic element of energy storage technology evaluation. Energy storage applications are different from battery applications in the fields of mobile communications, electronic products, and automobiles. The main difference is its large scale, large and centralized batteries, complex control, and huge investment. Once a safety problem occurs, it will cause huge losses. In recent years, many energy storage power station safety accidents have occurred worldwide. In July 2018, the economic loss caused by only one ternary lithium-ion battery energy storage station in the Yeongam Wind Power Park in South Korea was as high as 4.6 billion won. Safety is a system engineering. The causes of the above accidents not only include the thermal runaway of the energy storage battery, but also involve battery management systems. Systematic research is needed to establish an energy storage safety evaluation system. Therefore, safety must be the primary indicator for evaluating battery energy storage. On the one hand, the industry must strengthen the formulation of safety standards, and on the other hand, it must develop safer energy storage bodies and security control systems.
(2) Cost: The cost per kilowatt hour (for capacity-based energy storage application scenarios, the duration of continuous energy storage is not less than 4 h) and mileage cost (for power-based energy storage application scenarios, continuous energy storage The duration is 15-30 min), and the calculation is shown in equations (1) and (2). The cost and economic benefits of energy storage systems are important factors that determine whether they can be industrialized and scaled. Energy storage technology can only have an independent market position and become an indispensable part of the modern energy architecture if it achieves low cost on the basis of safety. Among the current battery energy storage technologies, the cost per kilowatt-hour of lithium iron phosphate batteries, all-vanadium flow batteries, sodium-sulfur batteries and lead storage batteries is 0.61 to 0.95 yuan/(kW·h), and the target cost for large-scale applications is 0.3 to 0.4 yuan. /(kW·h) There is still a gap.
(3) Technical performance: The basic performance of energy storage equipment that meets user needs, such as capacity, power, response time, cycle times, life, charge and discharge efficiency and other factors. At present, there are many application scenarios for energy storage, covering all aspects of power system generation, transmission, distribution, and use. Due to different roles, the requirements for energy storage equipment are also different. For example, from the perspective of power generation, energy storage application scenarios include six types of energy time shifting, capacity generating units, load tracking, system frequency modulation, reserve capacity, and grid connection of renewable energy. Energy time shift and capacity units play the role of peak shaving and valley filling, and have low requirements for charging and discharging power, time, annual operating frequency, and corresponding speed. Load tracking, system frequency modulation, and reserve capacity are typical power applications. In order to solve the problem of the slow speed of traditional energy generation, the energy storage system needs to have a fast response speed and high annual operating frequency. Renewable energy power generation has both power-based and energy-based applications. For photovoltaic, due to its discontinuity, it is necessary to store the excess electricity during the day and release it at night, which is a time shift of renewable energy. For wind power, due to its large fluctuations, it needs to be smoothed, so power-based applications are the mainstay.
(4) Environmental friendliness: low environmental load throughout the life cycle. For energy storage technology, on the one hand, it is necessary to reduce the damage to the environment during the construction and use of the energy storage system, and on the other hand, to do a good job in the recycling and reuse of materials in the energy storage system. Energy storage is a resource-intensive industry, and the carriers of energy storage are chemicals. Especially for battery energy storage, it involves multiple elements. However, the content of each element in the earths crust is different. For example, the content of cobalt in the earths crust is 0.001%, which is mostly associated with other mineral deposits, and the content is low. With the rapid increase of power batteries, consumption gradually increases. In battery energy storage technology, these precious metals are all recyclable. Therefore, improving the recovery rate of cobalt and simplifying the process flow in power batteries are also current research hotspots.
Aqueous lithium-ion battery cathode materials are mainly divided into four types: lithium manganese oxide, lithium cobalt oxide, lithium iron phosphate and ternary materials. Lithium manganese oxide compound has a spinel structure, a theoretical specific capacity of 148 mA·h/g, abundant resources, and low cost. It is currently the most widely used cathode material. Common anode materials are mainly alumina-oxygen compounds, polyanionic compounds, and other non-metallic materials such as silicon and tin. Due to the low capacity, poor cycle performance and low rate performance of vanadium oxy-type compounds, polyanionic compounds (such as lithium titanium phosphate) have attracted the attention of many researchers. At present, the exploration of electrode materials based on water-in-salt electrolyte system has made some progress, but it is far less abundant than traditional lithium-ion batteries and still needs further optimization.
The solid electrolyte interface (SEI) is critical to the cycling process of lithium-ion batteries. SEI film cannot be formed in traditional water-based lithium ion batteries, and battery cycle performance is poor. However, SEI was first observed in the "water in salt" electrolyte. According to theoretical calculations and molecular dynamics simulation results, high concentration of LiTFSI formed a large number of polymerized ion pairs, which increased the reduction potential of the anion, making the anion have It is possible to decompose and reduce to LiF under conditions higher than the hydrogen production potential to cover the surface of the negative electrode. This broadens the electrochemical window of the aqueous solution in terms of thermodynamics and kinetics, and inhibits the occurrence of hydrogen evolution. In addition, as a carrier supporting active materials and collecting current, current collectors are an important part of rechargeable metal ion batteries. Among them, metal mesh (such as stainless steel mesh and titanium mesh) is widely used in RAIB. However, during the charge/discharge cycle, the electrolysis of water and the corrosiveness of the aqueous electrolyte will affect the long cycle life of the current collector and the aqueous ion battery. Therefore, it is necessary to explore a new type of anti-corrosion current collector on the one hand, and on the other hand to construct a surface protective layer. For example, Yao Yan et al. reported that chromate conversion film aluminum foil used as a corrosion-resistant current collector in the cathode of water-based lithium-ion batteries has better cycle stability and higher coulombic efficiency, and can also inhibit oxygen escape at high potentials. Out.
2.2 Flow battery
The flow battery is mainly composed of a stack and two electrolyte storage tanks. Normally, the electrolyte is pumped from the storage tank to the inside of the stack, and the redox reaction occurs through the electrodes, where the chemical energy is converted into electrical energy (discharge), and vice versa (charge). Between the anode chamber and the cathode chamber is a diaphragm, which can selectively allow the cross-transport of inactive substances (such as H+, Cl-) to maintain electrical neutrality and electrolyte balance. The power density of a flow battery is determined by the size of the electrode and the number of batteries in the stack, while the energy density is determined by properties such as the concentration and volume of the electrolyte. Therefore, the flow battery can realize the independent design of power density and energy density. This feature makes the flow battery have a rich application scenario. For example, the all-vanadium flow battery has mature technology and has developed to the commercialization stage. It has high energy efficiency (>80%), long cycle life (>200,000 cycles), good safety, modular design, and high power density. , Suitable for large and medium-sized energy storage scenarios. In recent years, low-cost, high-energy density flow batteries have attracted more and more attention. On the one hand, they make up for the shortcomings of traditional all-vanadium flow batteries. On the other hand, they are also conducive to promoting flow batteries in special scenarios such as 5G and artificial intelligence. Applications.
Cost reduction is generally carried out from two aspects. ① Electrolyte. Use iron, manganese, zinc and other metals rich in the earths crust as active components of the electrolyte. As reported by Savinell et al., the working principle of the all-iron flow battery is shown in Figure 6(a). The poor performance of the traditional all-iron flow battery is improved by reversibly electroplating iron metal on suspended and flowing carbon particles. The working principle of the zinc-iodine flow battery reported by Li Xianfeng and others is shown in Figure 6(b). The active materials in the positive and negative electrolytes are KI and ZnBr2 respectively; or use carbon, nitrogen, oxygen, sulfur, etc. Composition of cheap organic matter, such as the alkaline quinone water-based flow battery reported by Aziz et al. Its working principle is shown in Figure 6(c). The system uses 2,6-dihydroxyanthraquinone and potassium ferrocyanide as the The negative and positive electrolytes pioneered the use of anthraquinones as electrolytes for flow batteries. ② Diaphragm. Choose low-cost membranes such as composite membranes and anion exchange membranes. As shown in Figure 6(d), the polyvinylidene fluoride (PVDF)/graphene composite nanoporous membrane reported by Wang Baoguo, etc., graphene can enhance the selectivity and conductivity of the membrane, by adding 0.15% (mass fraction) Graphene, the energy efficiency of the vanadium redox flow battery has increased by 13%; as shown in Figure 6(e), the PES/SPEEK composite porous membrane reported by Zhang Huamin and Li Xianfeng, with PES as the substrate, SPEEK can control the morphology of the membrane It can also provide negative charge. Applying the film to a low-cost zinc-iron flow battery system not only reduces the cost but also solves the problem of zinc dendrites, while improving battery performance.
image
Figure 6 (a) All-iron flow battery; (b) Zinc-iodine flow battery; (c) Alkaline organic flow battery; (d) Polyvinylidene fluoride/graphene composite nanoporous film; (e) Zinc Iron flow battery; (f) All liquid organic flow battery
The energy density of a flow battery is determined by the concentration of the active material, the number of transferred electrons, and the battery potential. Therefore, energy density can be improved from these three aspects. For example, the high energy density all-liquid organic flow battery reported by Xing Xueqi et al. The working principle is shown in Figure 6(f). The system uses active materials that are miscible with organic solvents. , So the solubility is relatively high; through the adjustment of the position of the active material functional group, the battery potential is expanded to 2.97 V. Although the active material of the system only transfers one charge, the battery energy density has reached 223 W·h/L, much higher than before The reported non-aqueous organic flow battery, and the coulombic efficiency and energy efficiency can reach 95% and 70%, respectively.
Flow batteries have already started in terms of industrialization, and are currently being applied in scenarios such as new energy plus flow battery energy storage, urban energy storage power stations, and energy storage frequency modulation. Energy storage body technology is expected to rise to a higher level in the near future. First of all, the power density of the stack technology has recently achieved a substantial increase. The National Energy Group Beijing Low-Carbon Clean Energy Research Institute and other units have increased the power density of the all-vanadium flow battery stack to more than 200 mW/cm2, which is higher than the current commercial Chemical products are increased by 1.0 to 1.2 times, effectively improving the utilization rate of core materials and reducing battery costs. Secondly, Tsinghua University and other units raised the working temperature of the vanadium electrolyte to 50 ℃, realized the air cooling of the energy storage system, and reduced the complexity of the system and the hardware investment cost. In addition, the Central Research Institute of the State Power Investment Corporation has prepared a new type of Fe/Cr flow battery high-power stack. If further experiments are successful, it will greatly reduce the energy storage cost of the flow battery.
2.3 Sodium Sulfur Battery
Sodium-based batteries, which belong to molten salt batteries, are a type of electrochemical energy storage device that uses molten salt as electrodes and/or electrolytes. At present, they mainly include sodium sulfur (Na-S) batteries and sodium metal halide (ZEBRA) batteries. The anodes of these two types of batteries are molten salt sodium, while the cathodes are molten sulfur and solid metal halides, and the solid electrolyte is β-alumina. Figure 7 is a schematic diagram of the working principle of a sodium-based battery. In order to keep the molten salt in a molten state and promote reaction kinetics, the operating temperature of molten salt batteries is relatively high (>300 ℃).
At present, industrial companies headed by Japan NGK have implemented and constructed a number of sodium-sulfur battery energy storage projects in Japan, the United States, the UAE and other countries. However, in September 2011, NGKs sodium-sulfur battery installed at the Mitsubishi Materials Tsukuba Plant in Ibaraki Prefecture, Japan caused a fire due to the leakage of the high-temperature melt on the top of the battery, which lasted for two weeks. This accident caused people to worry about the durability, cost and safety of battery materials brought about by the high temperature of sodium-sulfur batteries, which affected its further development. In recent years, a lot of research has been devoted to reducing the operating temperature of sodium-sulfur batteries. Holze et al. reported that polyacrylonitrile/sulfur composites are used as the cathode materials of room-temperature sodium-sulfur batteries. The initial specific capacity is about 655 mA·h/g, charging/discharging The efficiency is about 100%. Ahn et al. reported that the organic solvent tetraethylene glycol dimethyl ether is used as the electrolyte for the room temperature sodium-sulfur battery. Although the cathode component is dissolved in the liquid electrolyte, resulting in a rapid decline in battery capacity, a dense solid diaphragm such as β,,-Al2O3 can be used. Help solve such problems. Due to the high theoretical energy density, high charge and discharge energy efficiency, and long cycle life of sodium-sulfur batteries, sodium-sulfur batteries are still one of the choices for stationary applications and transportation application scenarios if they can solve the safety problem.
2.4 lead battery
Lead storage batteries mainly include lead-acid batteries and lead-carbon batteries. Lead-acid battery is a kind of storage battery that uses lead compound and metal lead as positive and negative electrode materials, and sulfuric acid solution as electrolyte. Lead-acid batteries have abundant raw materials, mature manufacturing processes, low prices, and safe and reliable performance. They have been used in many fields such as communications, transportation, and electricity. However, in large-scale energy storage and electric vehicle applications, the recycling of lead-acid batteries under high-rate partial charge state (HRPSOC) will cause irreversible sulfation of the negative electrode, which greatly reduces the service life of lead-acid batteries. The lead-carbon battery is a new type of battery formed by applying the activated carbon electrode material of a supercapacitor to a traditional lead-acid battery. The hybrid super battery shown in Figure 8, the negative electrode is composed of two parallel lead and carbon electrodes. Compared with traditional lead-acid batteries, the life span can be increased by an order of magnitude or more.
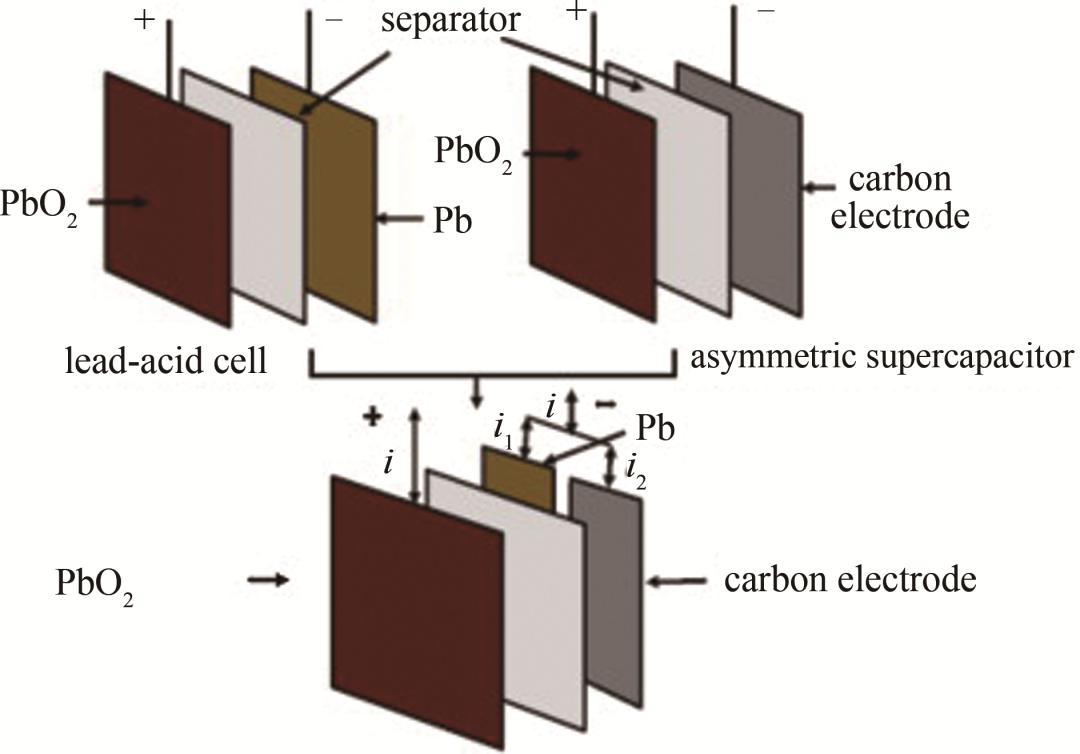
In recent years, domestic and foreign scholars have invested a lot of energy in the research and development of lead-carbon batteries. At present, the carbon materials used in lead-carbon batteries mainly include carbon black, activated carbon, graphite fiber, graphene, carbon nanotubes, etc. Different degrees of modification. Zhang Tianren and others reviewed the mechanism of carbon materials in lead-acid batteries, which are mainly divided into conductive mechanism, steric hindrance mechanism, parallel reaction mechanism and electric double layer energy storage mechanism. Although the addition of carbon materials has significantly improved the life of lead-acid batteries, there are still some problems, such as the type and amount of carbon materials that are not yet clear, the negative hydrogen evolution reaction shortens the cycle life of the battery, the process needs to be further optimized, and the cost of electricity needs to be further reduced. Wait.
Compared with other battery energy storage technologies, lead-acid batteries have been applied in the fields of hybrid electric vehicles, renewable energy access, peak shaving and valley filling, and smart microgrids due to their low cost and highly mature industrial level. With the gradual maturity of lead-carbon batteries, this energy storage technology will surely become one of the ideal energy storage technology choices.
3 Outlook and conclusion
The energy storage industry is going through a critical period of gradual maturity of energy storage technology and the gradual establishment of the energy storage market. During this period, opportunities and challenges coexist.
At present, the policies related to energy storage development need to be further rationalized. On the one hand, the Guiding Opinions on Promoting my countrys Energy Storage Technology and Industrial Development and the Implementation of theAction Plan for 2019-2020 issued at the national level have effectively promoted the Demonstration of energy projects. A number of local energy storage subsidy policies have brought opportunities to the energy storage market. On the other hand, the State Grid issued the Notice on Further Strict Control of Grid Investment and stated that the construction of grid-side electrochemical energy storage facilities shall not be carried out by means of investment, lease or contract energy management. The state issued the Notice of the National Development and Reform Commission on Relevant Matters Concerning the Reduction of General Industrial and Commercial Electricity Prices. The annual revenue of energy storage projects for general industrial and commercial users in Jiangsu has decreased by 4% to 8%, causing the consumer market to become cold. However, in the long run, with the gradual improvement of the power spot market, the scale of grid-side energy storage will gradually be channeled to the power-supply side. In the future, grid companies will serve as a platform or a mode to support access, which means new opportunities for energy storage.
At the technical level, battery energy storage technology should develop towards the goal of "high safety, low cost, long life, and environmental friendliness. ①Aqueous lithium-ion batteries have the possibility of further development. The development of electrode materials, electrolytes, additives, current collectors and other components needs to be overcome; the energy density needs to be increased from the current 25 W·h/kg to at least 50-100 W·h/kg to ensure its flexible configuration, the cost of electricity needs to be reduced to the level of traditional lithium-ion batteries without reducing the energy density. ②The all-vanadium redox flow battery is currently being demonstrated at the 100MW level, and the technical feasibility has been proven. With the advancement of manufacturing technology, the breakthrough of material technology, and the improvement of the domestic industrial chain, the cost per kilowatt-hour of vanadium redox battery is expected to fall below 0.7 yuan/(kW·h). In addition, new flow battery systems with high energy density (> 200 W·h/L), such as zinc iodine, zinc bromide, and organic flow batteries, have been reported and still need further development and verification. ③Safety is still the primary factor restricting sodium-sulfur batteries. The development of sodium-sulfur batteries at lower temperature or room temperature is a good choice. ④ Compared with other battery energy storage technologies, lead-carbon batteries have the advantages of low cost and mature technology. If the life is further optimized, the application prospects will be broader. ⑤Other battery energy storage technologies such as water-based metal ion batteries and metal-air batteries have recently emerged. If the right application scenarios are selected, they will become powerful competitors in the industry. Generally speaking, there is no battery energy storage technology that can meet all the needs of large-scale energy storage, and the future will be a situation where multiple technologies will develop together.
In addition, there is an urgent need to build a standard system for energy storage systems and an industrial process service model that meets user needs. However, the development trend of battery energy storage has been irreversible, and its role in the generation, transmission, distribution, transformation, and use of modern power grids has gradually manifested. The success of the United States, France, and the United Kingdom in the field of energy storage has provided China The development of energy provides a good reference. In addition, the early domestic practices in lithium-ion battery energy storage, flow battery energy storage and lead-acid battery energy storage have laid a solid foundation for the rapid development of battery energy storage.
Cite this article: Miao Ping, Yao Zhen, LEMMON John, etc.. Research progress and prospects of battery energy storage technology[J]. Energy Storage Science and Technology, 2020,09(03):670-678.
MIAO Ping,YAO Zhen,LEMMON John,et al.Current situations and prospects of energy storage batteries[J].Energy Storage Science and Technology,2020,09(03):670-678.
Author: Miao Ping (1963-), male, Ph.D., is mainly engaged in the research of new energy, hydrogen and energy storage fields such as, E-mail: ping.miao.c@chnenergy.com.cn
Corresponding author: Chen Yao main research directions for the flow battery energy storage, E-mail: zhen.yao.c@chnenergy.com.cn.
- Previous: China National Pharmac
- Next: A Rising 2D Star: Nove