ACS Nano: photothermal responsive conductive hydrogel for peripheral nerve repair
QQ Academic Group: 1092348845
Detailed
Biological tissues are soft, elastic, variable, reconfigurable, and renewable. The rapid development of flexible electronics has brought many advantages to bionic materials, such as stretchability, winding, curling, degradability, and self-healing properties. Therefore, they can be used in wearable electronics, artificial skin, personal health managers, medical implants, human-computer interaction interfaces, etc. Hydrophilic hydrogel is more suitable for the biological microenvironment of active tissues, making it a rational bioelectronic material.
Peripheral nerve damage often leads to nerve conduction disorders, chronic pain, paralysis and even disability. Autologous nerve transplantation is a common method to repair peripheral nerves. At present, there are the following problems that plague clinical repair of peripheral nerves: shortage of donors, need for several operations, prolonged hypertension, synaptic aplasia, risk of postoperative painful neuroma formation, especially for severe nerves that are difficult to suture with severed ends Interrupted.
Nerve tissue engineering is to repair the functions of motor and sensory nerves. Use natural materials or synthetic materials as implanted nerve catheters or stents for the growth of nerve cells. Clinically, it is hoped that a graft with effective and rapid repair will replace autologous nerve graft. Conductive hydrogels have become the first choice because they combine the biocompatibility of hydrogels and the advantages of conductive polymers that can conduct electrical signal transmission in nerve tissue, and can also use electrical signals to promote nerve cell regeneration.
Hydrogel simulating active tissue has the following advantages: high toughness, effective self-healing ability and sensitivity to physiological environment. The researchers designed a photothermal responsive stretchable conductive hydrogel with good peripheral nerve function. When mechanically extended, the hydrogel has conduction tolerance, so it is suitable for suture of accidental stretching of nerves during exercise. It is suitable for the repair of severe peripheral nerve damage, especially nerve loss over 10mm. When irradiated with near-infrared light, the conductivity of the hydrogel increases (Figure 1).
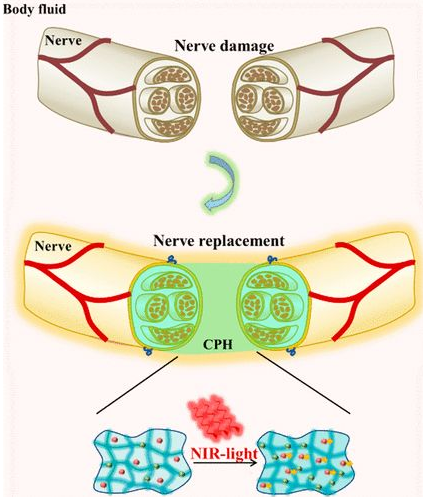
Experimental design: Conductive polymer hydrogel with high flexibility and near-infrared light response is used to repair peripheral nerve injury
The development of modern flexible electronic technology has used bioelectronic materials as artificial tissues in the body. Hydrogel is similar to nerve tissue, and functionalized hydrogel has become a cutting-edge candidate material for bioelectronics. Peripheral nerve injury often leads to paralysis, chronic pain, neurological dysfunction and even disability. Because its damage affects the electrical signal conduction between the brain and the body. Researchers have developed a photosensitive stretchable conductive polymer (CPH) for artificial nerve research. Near-infrared light can promote the conductivity of CPH, thereby promoting the conduction of bioelectric signals. When CPH is mechanically extended, it can still maintain conductivity, so it can withstand accidental stretching of nerve tissue during exercise. CPH can be used as a good graft for severe nerve injury, especially for peripheral nerve loss longer than 10mm.
CPH preparation: By copolymerizing polyaniline (PANI) and polyacrylamide (PAM), a flexible conductive hydrogel with excellent electrical conductivity, mechanical properties, and biocompatibility, similar to that of nerve tissue, is prepared. The amide bond of PAM can interact with aniline molecules, which can adsorb in situ and induce aniline polymerization to form a transparent PAM/PANI conductive gel (1A). The CPH was freeze-dried and photographed by electron microscope, showing a porous structure. CPH can conduct electricity when there is no or different strain tension. Under near-infrared light irradiation, PANI can enhance the bioelectrical signals of peripheral nerves and play an important role in repairing and/or replacing damaged peripheral nerves.
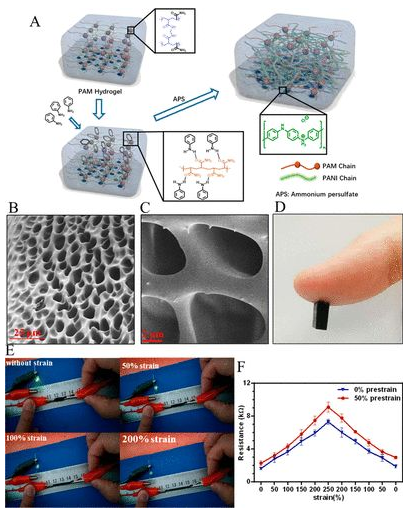
Figure 1 Preparation of CPH
CHP physical and electrical properties: The addition of PANI makes the resistivity of CPH significantly higher than PAM, higher than the original conductive hydrogel ITO, and higher than nerve tissue, so it can be used for nerve tissue repair (2A). Moreover, the electrical activity of CPH in PBS can be detected by electrochemical impedance, and the Nyquist curves of PAM and CPH are quasi-semi-circular in the high frequency region, indicating that both PAM and CPH have the ability of charge transfer (2B). Compared with PAM, CPH has a lower charge transfer resistance. The swelling of PAM in PBS is 4400%, and CPH is 2200% (2C). Although it is reduced, it can still meet the needs of nerve stretching during exercise. The storage modulus is lower than the loss modulus (2D), indicating that CPH is a stable elastomer. The CPH modulus of elasticity is 350Pa, which is suitable for biological transplantation. Finite element analysis of the applicability of CPH to replace damaged sciatic nerve bioelectric signal conduction. Myelin can isolate nerves from conductive body fluids and promote the transmission of bioelectric signals. CPH can conduct the electrical signal of the nerve after truncation, and the loss of myelin structure makes the electrical signal partly lost, but does not affect the signal transmission (2E). Because PANI has near-infrared light response characteristics, researchers consider whether near-infrared light can be used to promote signal transduction in biological points. Under near-infrared light irradiation, the loss of CPH bioelectric signal is reduced (2F). The electron transfer of the porous structure makes the CPH exhibit a current response. After CPH is irradiated for 60ms, the current increases from 1.95 nA to 2.3 nA, which proves that CPH has the characteristics of near infrared light response (2G). Finite element analysis also shows the same result, the current can be increased by 60%, that is, the resistance is reduced by 40% (2H). Under different stretching conditions under NIR light irradiation, the resistivity generally showed a downward trend. After removing the NIR light irradiation, the resistivity returned to the original level (2I).
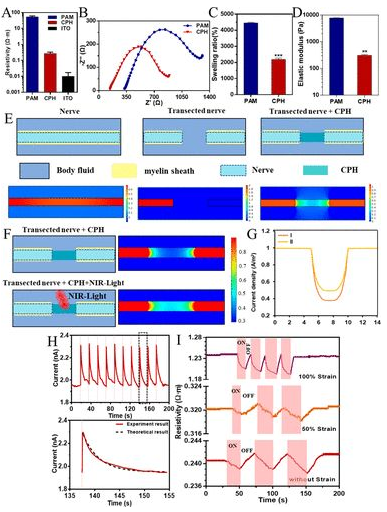
Figure 2 Physical and electrical properties of CPH
In vitro toad nerve detection: to isolate toad sciatic nerve, in vitro detection of CPH electrical signal transmission. As shown in Figure 3A and 3B, stimulation of the sciatic nerve can cause the gastrocnemius muscle to contract. As CPH increases, gastrocnemius contraction increases (3D). The conduction time of CPH is similar to that of native nerve, suggesting that CPH can be used to repair peripheral nerve damage (3E). The contraction tension of gastrocnemius muscle decreases with the increase of CPH stretch length. When CPH is stretched to 1.3 times, gastrocnemius muscle contraction tension retains more than 90% (3F). The researchers found that the gastrocnemius muscle under near-infrared light (915 nm) produces significantly higher tension than under dark light or blue light (Figure 3G). In addition, since near-infrared light has good penetration into tissues, it will be possible to use near-infrared light to enhance nerve conduction and replace damaged nerves in the body.
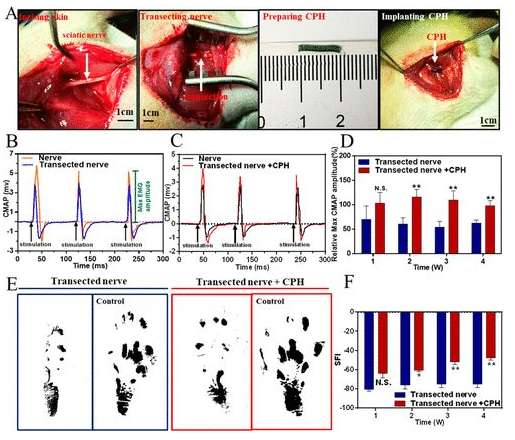
Figure 3 CPH replacement of toad sciatic nerve in vitro
Rat model sciatic nerve repair: Find the rat sciatic nerve and implant 1CM CPH into the injury model (4A). When the sciatic nerve was stimulated with a single-phase electrical waveform (5 millisecond single pulse), the peak symmetry of CMAPs in the transection group was significantly different from that of healthy nerves. In contrast, the peak symmetry of the CPH replacement group is similar to that of healthy nerves, indicating that CPH can replace injured nerves and achieve bioelectric signal transduction (4B, 4C). In addition, the researchers also analyzed the maximum amplitude of CMAPs at different times. In all stages of recovery, especially after 2 and 4 weeks after surgery, the activation of the tibial anterior muscle in the CPH replacement group was significantly higher than that in the transection group (4D). Gait experiments showed that the CPH replacement group was better than the transection group (4E, 4F).
The results of HE staining showed that the CPH replacement group and the transection group had less inflammation and fibrosis. Toluidine blue staining showed that the myelin sheath of the CPH replacement group was larger and thicker than that of the transection group (5A, B). The increase of GFAP in the tissue is a sign signal of the nervous systems response to injury, which is beneficial to the repair of nerve injury. One month after the replacement of CPH, the expression level of GFAP was higher than that of the transection group, which proved that CPH has good biological safety, and CPH may cause peripheral nerve repair (5C). High levels of CGRP are usually expressed at the proximal stump of the injury site. The expression level of CGRP is similar to that of the sciatic nerve group, but lower than that of the transection nerve group, indicating that CPH replacement has no obvious stimulating effect on the injured nerve tissue (5D).
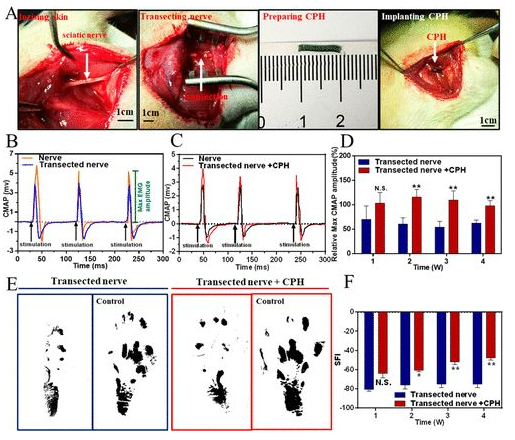
Figure 4 Surgical implantation
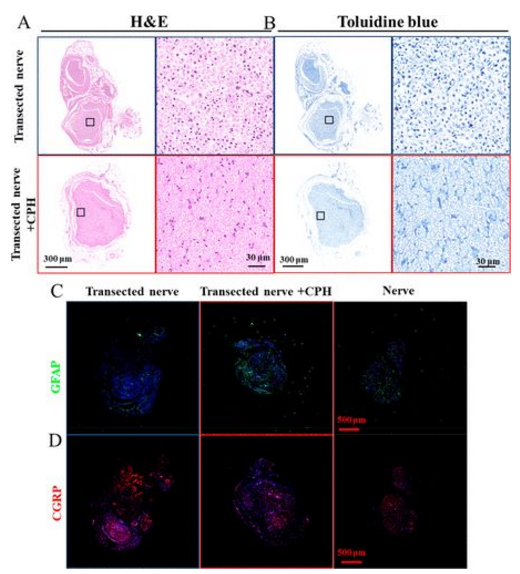
Figure 5 Biocompatibility of CPH in vivo: (A, B) HE staining of neurotoluidine blue staining in contact with CPH or transected nerve; (C, D) immunofluorescence images of GFAP and CGRP
In short, the researchers used PAM and PANI to copolymerize to form CHP with good electrical conductivity, biocompatibility, and mechanical properties similar to those of nerve tissue. The electrophysiological test of toad nerves in vitro has proved its good performance; in vivo data shows that transplantation of CPH can replace the loss of sciatic nerve. It is suggested that CPH can be used as a potential biomaterial to replace damaged peripheral nerves in tissue engineering.
Peripheral nerve damage often leads to nerve conduction disorders, chronic pain, paralysis and even disability. Autologous nerve transplantation is a common method to repair peripheral nerves. At present, there are the following problems that plague clinical repair of peripheral nerves: shortage of donors, need for several operations, prolonged hypertension, synaptic aplasia, risk of postoperative painful neuroma formation, especially for severe nerves that are difficult to suture with severed ends Interrupted.
Nerve tissue engineering is to repair the functions of motor and sensory nerves. Use natural materials or synthetic materials as implanted nerve catheters or stents for the growth of nerve cells. Clinically, it is hoped that a graft with effective and rapid repair will replace autologous nerve graft. Conductive hydrogels have become the first choice because they combine the biocompatibility of hydrogels and the advantages of conductive polymers that can conduct electrical signal transmission in nerve tissue, and can also use electrical signals to promote nerve cell regeneration.
Hydrogel simulating active tissue has the following advantages: high toughness, effective self-healing ability and sensitivity to physiological environment. The researchers designed a photothermal responsive stretchable conductive hydrogel with good peripheral nerve function. When mechanically extended, the hydrogel has conduction tolerance, so it is suitable for suture of accidental stretching of nerves during exercise. It is suitable for the repair of severe peripheral nerve damage, especially nerve loss over 10mm. When irradiated with near-infrared light, the conductivity of the hydrogel increases (Figure 1).
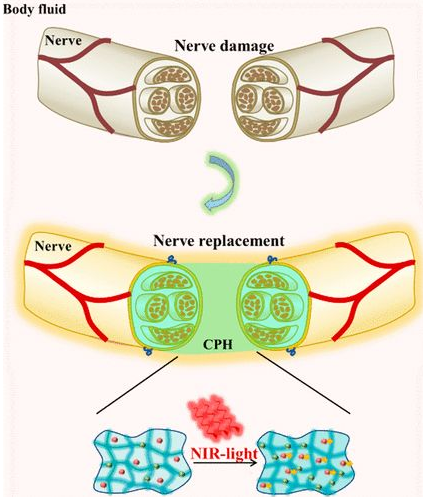
Experimental design: Conductive polymer hydrogel with high flexibility and near-infrared light response is used to repair peripheral nerve injury
The development of modern flexible electronic technology has used bioelectronic materials as artificial tissues in the body. Hydrogel is similar to nerve tissue, and functionalized hydrogel has become a cutting-edge candidate material for bioelectronics. Peripheral nerve injury often leads to paralysis, chronic pain, neurological dysfunction and even disability. Because its damage affects the electrical signal conduction between the brain and the body. Researchers have developed a photosensitive stretchable conductive polymer (CPH) for artificial nerve research. Near-infrared light can promote the conductivity of CPH, thereby promoting the conduction of bioelectric signals. When CPH is mechanically extended, it can still maintain conductivity, so it can withstand accidental stretching of nerve tissue during exercise. CPH can be used as a good graft for severe nerve injury, especially for peripheral nerve loss longer than 10mm.
CPH preparation: By copolymerizing polyaniline (PANI) and polyacrylamide (PAM), a flexible conductive hydrogel with excellent electrical conductivity, mechanical properties, and biocompatibility, similar to that of nerve tissue, is prepared. The amide bond of PAM can interact with aniline molecules, which can adsorb in situ and induce aniline polymerization to form a transparent PAM/PANI conductive gel (1A). The CPH was freeze-dried and photographed by electron microscope, showing a porous structure. CPH can conduct electricity when there is no or different strain tension. Under near-infrared light irradiation, PANI can enhance the bioelectrical signals of peripheral nerves and play an important role in repairing and/or replacing damaged peripheral nerves.
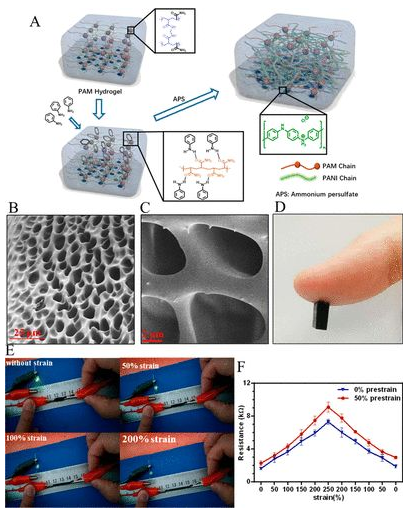
Figure 1 Preparation of CPH
CHP physical and electrical properties: The addition of PANI makes the resistivity of CPH significantly higher than PAM, higher than the original conductive hydrogel ITO, and higher than nerve tissue, so it can be used for nerve tissue repair (2A). Moreover, the electrical activity of CPH in PBS can be detected by electrochemical impedance, and the Nyquist curves of PAM and CPH are quasi-semi-circular in the high frequency region, indicating that both PAM and CPH have the ability of charge transfer (2B). Compared with PAM, CPH has a lower charge transfer resistance. The swelling of PAM in PBS is 4400%, and CPH is 2200% (2C). Although it is reduced, it can still meet the needs of nerve stretching during exercise. The storage modulus is lower than the loss modulus (2D), indicating that CPH is a stable elastomer. The CPH modulus of elasticity is 350Pa, which is suitable for biological transplantation. Finite element analysis of the applicability of CPH to replace damaged sciatic nerve bioelectric signal conduction. Myelin can isolate nerves from conductive body fluids and promote the transmission of bioelectric signals. CPH can conduct the electrical signal of the nerve after truncation, and the loss of myelin structure makes the electrical signal partly lost, but does not affect the signal transmission (2E). Because PANI has near-infrared light response characteristics, researchers consider whether near-infrared light can be used to promote signal transduction in biological points. Under near-infrared light irradiation, the loss of CPH bioelectric signal is reduced (2F). The electron transfer of the porous structure makes the CPH exhibit a current response. After CPH is irradiated for 60ms, the current increases from 1.95 nA to 2.3 nA, which proves that CPH has the characteristics of near infrared light response (2G). Finite element analysis also shows the same result, the current can be increased by 60%, that is, the resistance is reduced by 40% (2H). Under different stretching conditions under NIR light irradiation, the resistivity generally showed a downward trend. After removing the NIR light irradiation, the resistivity returned to the original level (2I).
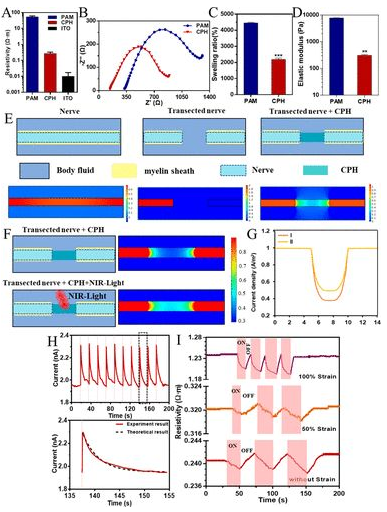
Figure 2 Physical and electrical properties of CPH
In vitro toad nerve detection: to isolate toad sciatic nerve, in vitro detection of CPH electrical signal transmission. As shown in Figure 3A and 3B, stimulation of the sciatic nerve can cause the gastrocnemius muscle to contract. As CPH increases, gastrocnemius contraction increases (3D). The conduction time of CPH is similar to that of native nerve, suggesting that CPH can be used to repair peripheral nerve damage (3E). The contraction tension of gastrocnemius muscle decreases with the increase of CPH stretch length. When CPH is stretched to 1.3 times, gastrocnemius muscle contraction tension retains more than 90% (3F). The researchers found that the gastrocnemius muscle under near-infrared light (915 nm) produces significantly higher tension than under dark light or blue light (Figure 3G). In addition, since near-infrared light has good penetration into tissues, it will be possible to use near-infrared light to enhance nerve conduction and replace damaged nerves in the body.
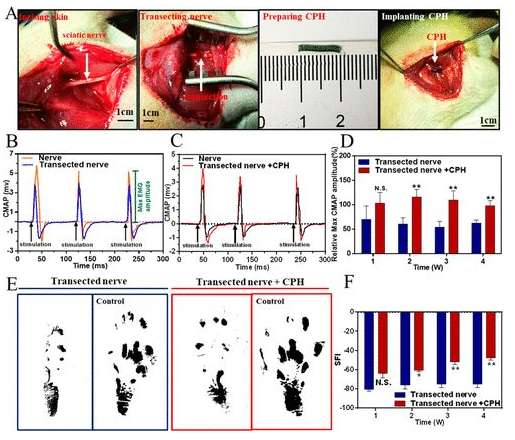
Figure 3 CPH replacement of toad sciatic nerve in vitro
Rat model sciatic nerve repair: Find the rat sciatic nerve and implant 1CM CPH into the injury model (4A). When the sciatic nerve was stimulated with a single-phase electrical waveform (5 millisecond single pulse), the peak symmetry of CMAPs in the transection group was significantly different from that of healthy nerves. In contrast, the peak symmetry of the CPH replacement group is similar to that of healthy nerves, indicating that CPH can replace injured nerves and achieve bioelectric signal transduction (4B, 4C). In addition, the researchers also analyzed the maximum amplitude of CMAPs at different times. In all stages of recovery, especially after 2 and 4 weeks after surgery, the activation of the tibial anterior muscle in the CPH replacement group was significantly higher than that in the transection group (4D). Gait experiments showed that the CPH replacement group was better than the transection group (4E, 4F).
The results of HE staining showed that the CPH replacement group and the transection group had less inflammation and fibrosis. Toluidine blue staining showed that the myelin sheath of the CPH replacement group was larger and thicker than that of the transection group (5A, B). The increase of GFAP in the tissue is a sign signal of the nervous systems response to injury, which is beneficial to the repair of nerve injury. One month after the replacement of CPH, the expression level of GFAP was higher than that of the transection group, which proved that CPH has good biological safety, and CPH may cause peripheral nerve repair (5C). High levels of CGRP are usually expressed at the proximal stump of the injury site. The expression level of CGRP is similar to that of the sciatic nerve group, but lower than that of the transection nerve group, indicating that CPH replacement has no obvious stimulating effect on the injured nerve tissue (5D).
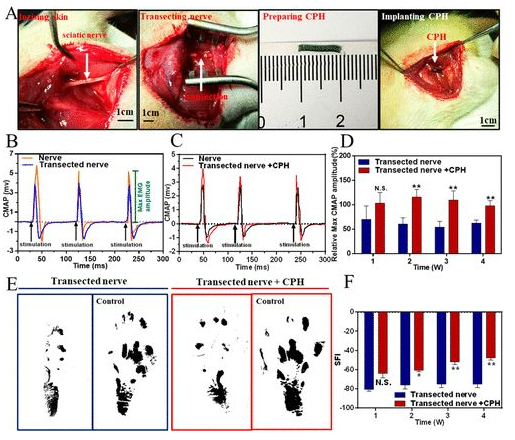
Figure 4 Surgical implantation
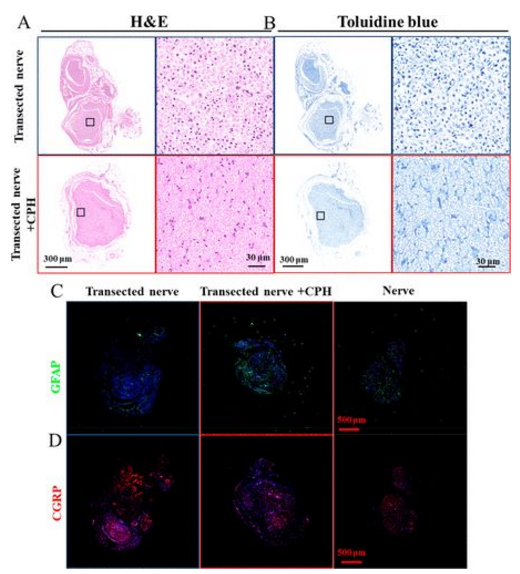
Figure 5 Biocompatibility of CPH in vivo: (A, B) HE staining of neurotoluidine blue staining in contact with CPH or transected nerve; (C, D) immunofluorescence images of GFAP and CGRP
In short, the researchers used PAM and PANI to copolymerize to form CHP with good electrical conductivity, biocompatibility, and mechanical properties similar to those of nerve tissue. The electrophysiological test of toad nerves in vitro has proved its good performance; in vivo data shows that transplantation of CPH can replace the loss of sciatic nerve. It is suggested that CPH can be used as a potential biomaterial to replace damaged peripheral nerves in tissue engineering.
- Previous: Analytical Chemistry:
- Next: A Rising 2D Star: Nove