Tsinghua Angew: Atomic Scale Control! Cobalt single-atom catalyst derived from MOFs
QQ Academic Group: 1092348845
Detailed
Commercial applications of energy conversion devices such as fuel cells and metal-air batteries rely heavily on efficient and cost-effective catalysts to drive electrochemical oxygen reduction reactions (ORR). Therefore, as a substitute for expensive and scarce Pt-based materials, the exploration and development of efficient and durable non-noble metal catalysts has attracted extensive research attention. Metal organic frameworks (MOFs) are a class of crystalline porous materials with a rich porous structure, excellent designability and adjustable functionality, and have been considered as templates for ORR electrocatalysts based on metal nitrogen carbon (MNC). Although many efforts have been devoted to the engineering design of MOF-derived M-N-C materials, their ORR catalytic performance is not enough to be a substitute for Pt-based electrocatalysts. One of the main reasons is that the heterogeneity of the composition and structure of the M-N-C catalyst hinders the identification of accurate active centers and the further improvement of catalytic performance.
To this end, researchers from Tsinghua University and other units recently published an article entitled "Atomic-level modulation of electronic density of metal-organic frameworks-derived Co single-atom sites to enhance oxygen reduction performance" on Angew Chem Int Ed. They combined density functional theory (DFT) calculations and electrochemical analysis to prove the correlation between the electron density induced by the atomic configuration of the single-atom Co active site and the ORR performance. Under the guidance of DFT calculations, design and synthesis The MOFs-derived Co single-atom catalyst has the best Co1-N3PS active part incorporated into hollow carbon polyhedrons (Co1-N3PS / HC).
Link to the paper: https://doi.org/10.1002/anie.202012798
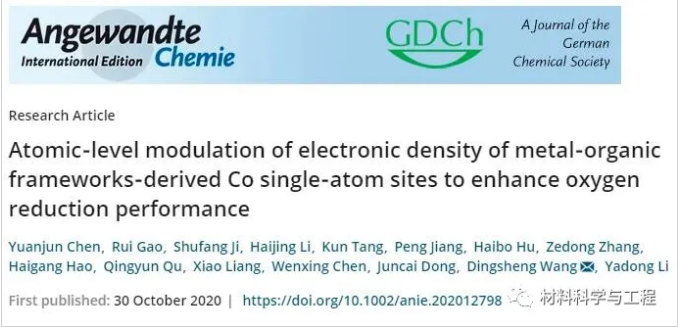
The study found that, as predicted, Co1N3PS/HC has excellent alkaline ORR activity, ORR kinetics, record-breaking dynamic current density and an ultra-low Tafel slope of 31 mV dec-1, with a half-wave potential of 0.920 V, Almost all non-noble metal ORR electrocatalysts surpassed Pt/C. Even in more challenging acidic media, the ORR kinetics of Co1-N3PS/HC still exceeds that of Pt/C. This work provides an analysis of the relationship between the atomic-level active site electron density and catalytic performance, and promotes the rational design of high-efficiency catalysts.
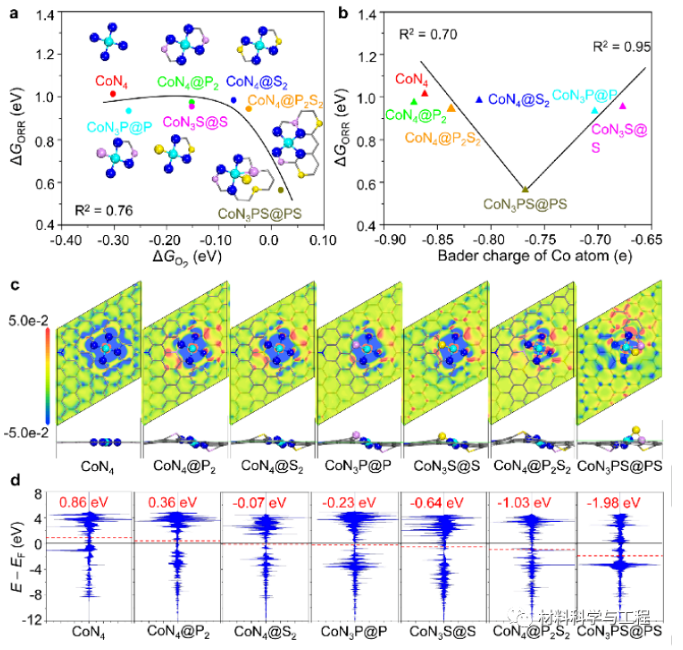
Figure 1. DFT study of ORR activity on different single-atom cobalt catalysts: (a) When URHE = 1.23 V, the linear relationship between ORR activity (ie overpotential) and O2 * adsorption energy (∆GO2); In the model structure, the Co, N, P and S atoms are cyan, blue, pink and yellow, and the carbon layer is a gray rod. (B) For the Bader charge of the Co atom in the active center, the ORR reverse volcanic overpotential, and the negative value indicates the electron donor. (C) In the seven catalyst models, the charge density of Co atoms is different. Red and blue indicate the increase and decrease of electrons, respectively. (D) Part of the state density (pDOS) of Co atoms in the model, with red fonts and lines inserted in the center of the d zone
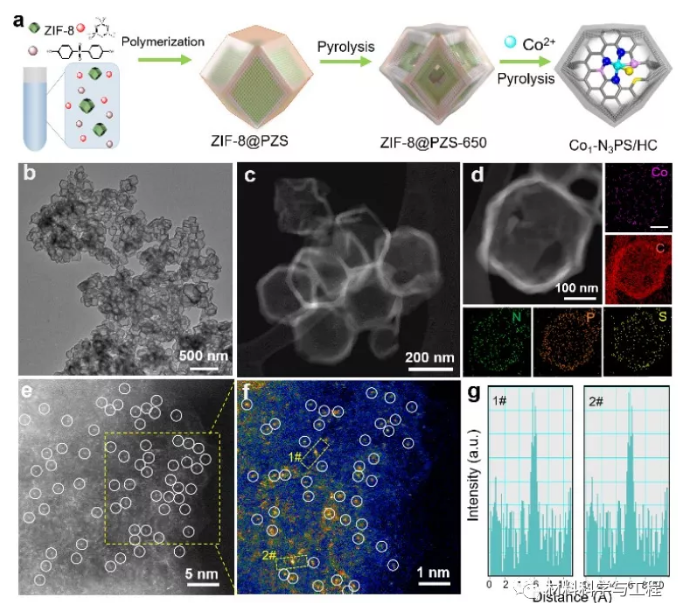
Figure 2. Synthesis and structural characterization of Co1-N3PS/HC. (A) Synthetic scheme road map of Co1-N3PS/HC. (B) TEM and (c) HAADF-STEM images of Co1-N3PS/HC. (D) The enlarged HAADF-STEM image and the corresponding EDS mapping diagram of Co1-N3PS/HC. (E) AC HAADF-STEM image and (f) Co1-N3PS/HC magnified intensity image. (G) Schematic diagram of the intensity distribution of Co1N3PS/HC obtained in the dotted rectangular area 1# and 2# in the figure f.
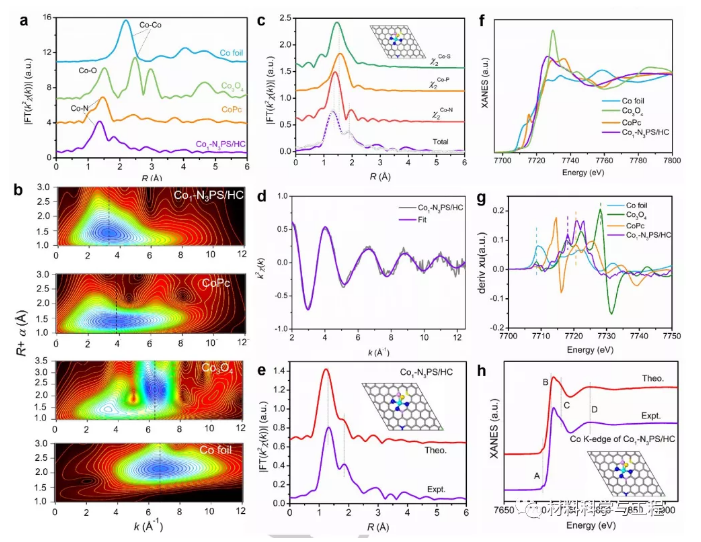
Figure 3. Atomic structure analysis of Co1-N3PS / HC. (A) Co K edge FT-EXAFS spectra of Co1-N3PS/HC and reference samples. (B) Co1-N3PS/HC and reference sample Co K edge WT-EXAFS contour map. (C) CoK edge EXAFS fitting analysis diagram of Co1-N3PS/HC in R space and (d) k space. (E) Compare the experimental FT-EXAFS spectrum (purple line) of Co1-N3PS/HC with the theoretically simulated FT-EXAFS spectrum (red line) based on the DFT model of the illustration. (F) Co K edge XANES spectrum and (g) Co1-N3PS/HC and the first derivative curve of the reference sample. (H) The DFT model based on the illustration compares the experimental XANES spectrum (purple line) of Co1-N3PS/HC and the comparison between the theoretically simulated XANES spectrum (red line).
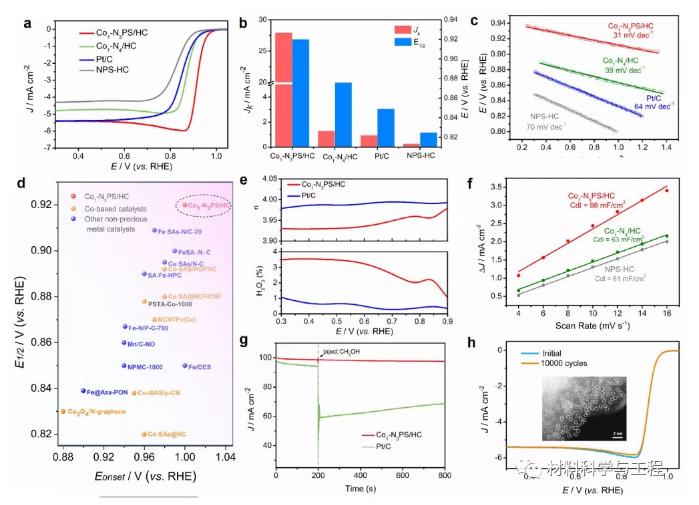
Figure 4. Electrocatalytic alkaline ORR performance of Co1-N3PS/HC. (A) Schematic diagram of the ORR polarization curve of Co1-N3PS/HC and reference catalyst in 0.1 M KOH. (B) Compare the Jk and E1/2 of Co1-N3PS/HC and the reference catalyst at 0.90 V. (C) Tafel diagram of Co1-N3PS/HC and reference catalyst. (D) Comparison of Co1-N3PS/HC in Table S4 with the previously reported Eonset and E1/2 of Co-based catalysts and other non-noble metal catalysts. (E) Schematic diagram of the electron transfer number of Co1-N3PS/HC (top) and H2O2 yield. (F) Electrochemical double layer capacitance (Cdl) of Co1-N3PS/HC and reference catalyst. (G) The tolerance curve of Co1N3PS/HC to methanol and the tolerance of 20% Pt/C at 0.70 V (inject methanol into 0.1 M KOH solution in 200 s). (H) The ORR polarization curves of Co1-N3PS/HC before and after 10,000 potential cycles (the inset is the AC HAADF-STEM image of Co1-N3PS/HC after durability test).
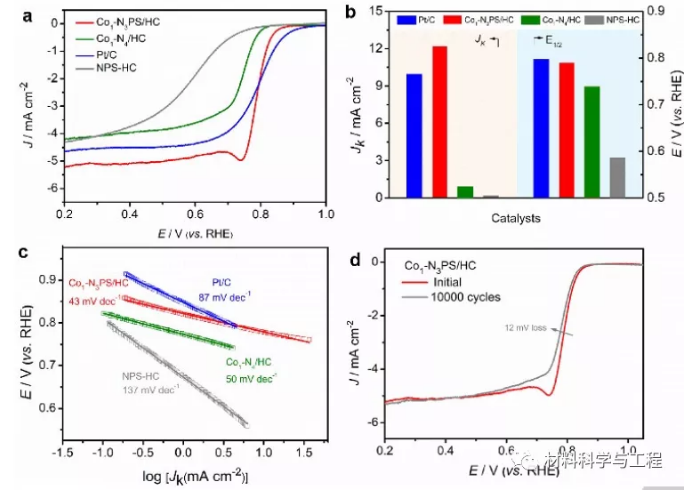
Figure 5. Electrocatalytic acid ORR performance of Co1-N3PS/HC. (A) Schematic diagram of ORR polarization curve of Co1-N3PS/HC and reference catalyst in 0.5 M H2SO4. (B) Comparison of the Jk and E1/2 schematic diagrams of Co1-N3PS/HC and the reference catalyst at 0.775 V. (C) Tafel diagram of Co1-N3PS/HC and reference catalyst. (D) Schematic diagram of the ORR polarization curve before and after 10,000 potential cycles of Co 1-N3PS/HC in 0.5 M H2SO4.
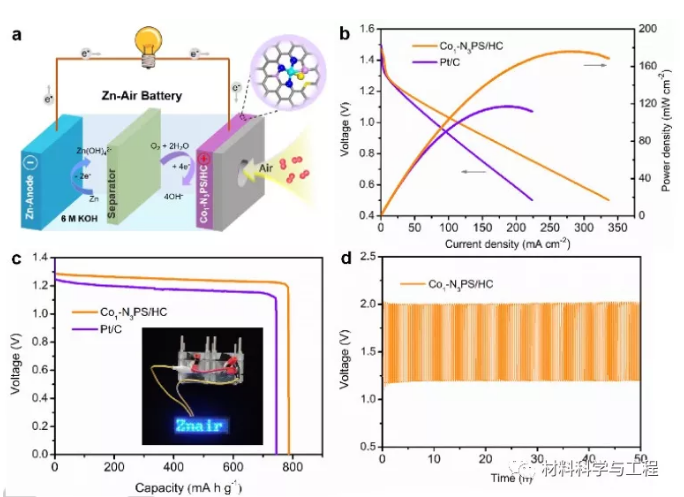
Figure 6. Co1-N3PS / HC zinc-air battery performance. (A) Schematic diagram of zinc-air battery. (B) The discharge polarization curve and power density diagram of zinc-air battery based on Co1-N3PS/HC and Pt/C. (C) The specific discharge capacity curve of Co1-N3PS/HC and Pt/C zinc-air batteries at 10 mA cm-2. (D) Constant current charge-discharge cycle curve of Co1-N3PS/HC-based zinc-air battery.
This paper proves that the ORR performance of the Co single-atom catalyst can be adjusted and optimized by accurately controlling the atomic configuration of the active site based on DFT calculation and electrochemical analysis. Guided by DFT calculations, a two-step pyrolysis strategy was developed to prepare a single-atom Co site catalyst with optimized CoN3PS active part. The atomic configuration of Co1-N3PS/HC has been confirmed by a series of advanced characterization techniques, including XPS analysis, AC HAADF-STEM and XAFS measurement. Co1-N3PS/HC shows high efficiency ORR reactivity and excellent ORR kinetics in both alkaline and acidic media, as well as good methanol tolerance and excellent stability, which is better than the commercially available Pt/C and large Most known non-noble metal electrocatalysts.
In addition, the zinc-air battery based on Co1N3PS/HC has excellent battery performance and long-term charge/discharge durability, proving its huge potential application prospects. According to the designed control experiment and DFT calculation, this paper confirms that the optimal electron density of the atomically dispersed Co active center caused by the unique coordinated coordination of N, P and S atoms plays a key role in the significant improvement of ORR performance. This work has improved the scientific understanding of the relationship between atomic site electron density and ORR performance, which will provide a new method for rational design and optimization of catalysts. (Text: SSC)
To this end, researchers from Tsinghua University and other units recently published an article entitled "Atomic-level modulation of electronic density of metal-organic frameworks-derived Co single-atom sites to enhance oxygen reduction performance" on Angew Chem Int Ed. They combined density functional theory (DFT) calculations and electrochemical analysis to prove the correlation between the electron density induced by the atomic configuration of the single-atom Co active site and the ORR performance. Under the guidance of DFT calculations, design and synthesis The MOFs-derived Co single-atom catalyst has the best Co1-N3PS active part incorporated into hollow carbon polyhedrons (Co1-N3PS / HC).
Link to the paper: https://doi.org/10.1002/anie.202012798
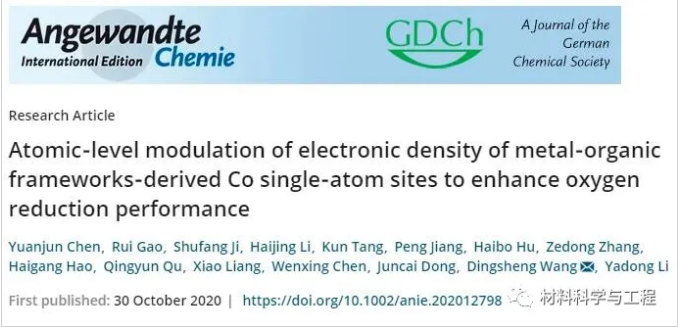
The study found that, as predicted, Co1N3PS/HC has excellent alkaline ORR activity, ORR kinetics, record-breaking dynamic current density and an ultra-low Tafel slope of 31 mV dec-1, with a half-wave potential of 0.920 V, Almost all non-noble metal ORR electrocatalysts surpassed Pt/C. Even in more challenging acidic media, the ORR kinetics of Co1-N3PS/HC still exceeds that of Pt/C. This work provides an analysis of the relationship between the atomic-level active site electron density and catalytic performance, and promotes the rational design of high-efficiency catalysts.
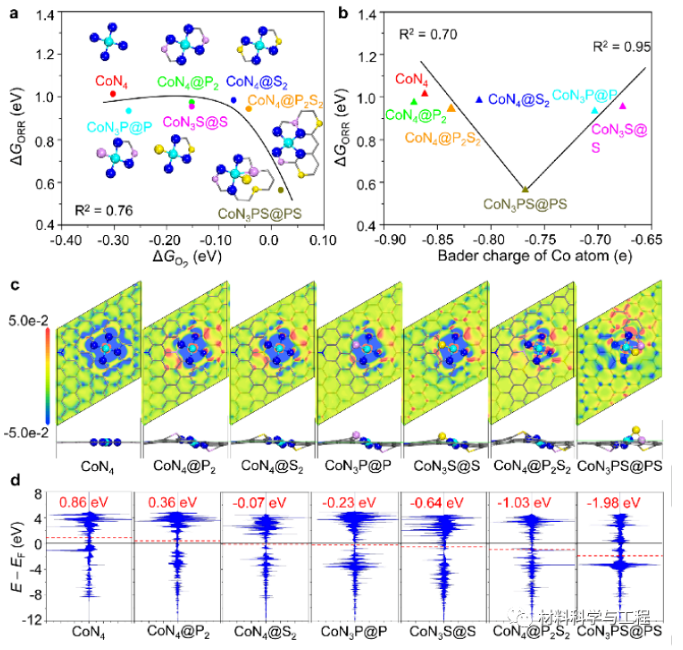
Figure 1. DFT study of ORR activity on different single-atom cobalt catalysts: (a) When URHE = 1.23 V, the linear relationship between ORR activity (ie overpotential) and O2 * adsorption energy (∆GO2); In the model structure, the Co, N, P and S atoms are cyan, blue, pink and yellow, and the carbon layer is a gray rod. (B) For the Bader charge of the Co atom in the active center, the ORR reverse volcanic overpotential, and the negative value indicates the electron donor. (C) In the seven catalyst models, the charge density of Co atoms is different. Red and blue indicate the increase and decrease of electrons, respectively. (D) Part of the state density (pDOS) of Co atoms in the model, with red fonts and lines inserted in the center of the d zone
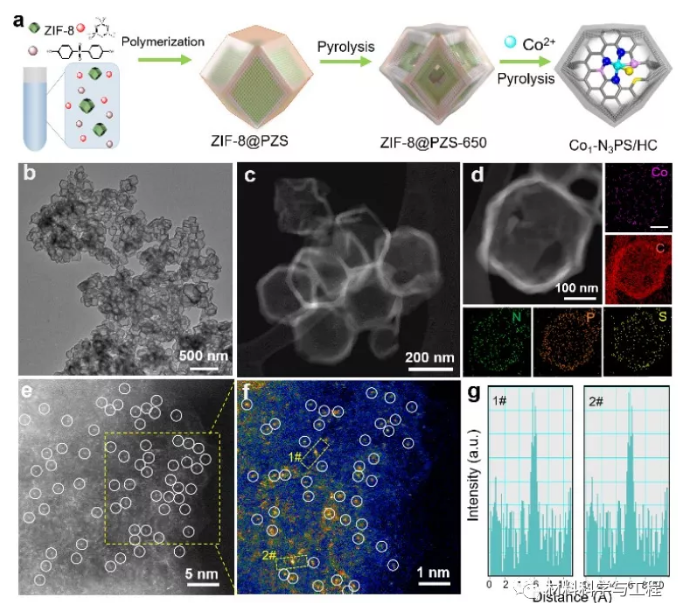
Figure 2. Synthesis and structural characterization of Co1-N3PS/HC. (A) Synthetic scheme road map of Co1-N3PS/HC. (B) TEM and (c) HAADF-STEM images of Co1-N3PS/HC. (D) The enlarged HAADF-STEM image and the corresponding EDS mapping diagram of Co1-N3PS/HC. (E) AC HAADF-STEM image and (f) Co1-N3PS/HC magnified intensity image. (G) Schematic diagram of the intensity distribution of Co1N3PS/HC obtained in the dotted rectangular area 1# and 2# in the figure f.
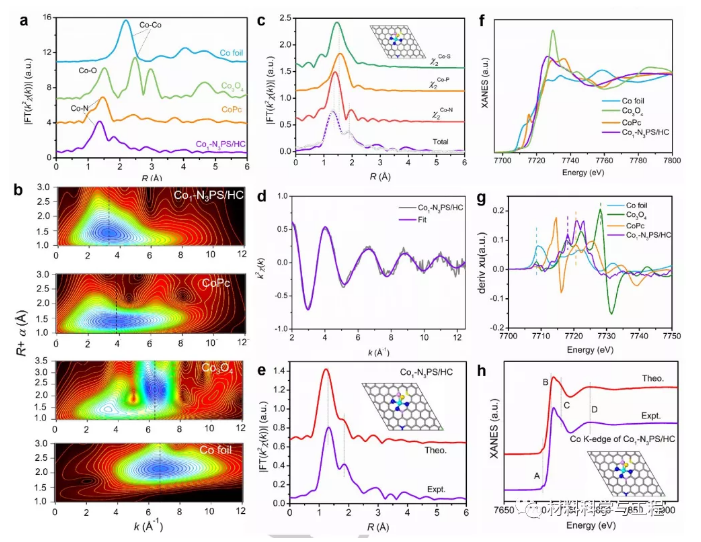
Figure 3. Atomic structure analysis of Co1-N3PS / HC. (A) Co K edge FT-EXAFS spectra of Co1-N3PS/HC and reference samples. (B) Co1-N3PS/HC and reference sample Co K edge WT-EXAFS contour map. (C) CoK edge EXAFS fitting analysis diagram of Co1-N3PS/HC in R space and (d) k space. (E) Compare the experimental FT-EXAFS spectrum (purple line) of Co1-N3PS/HC with the theoretically simulated FT-EXAFS spectrum (red line) based on the DFT model of the illustration. (F) Co K edge XANES spectrum and (g) Co1-N3PS/HC and the first derivative curve of the reference sample. (H) The DFT model based on the illustration compares the experimental XANES spectrum (purple line) of Co1-N3PS/HC and the comparison between the theoretically simulated XANES spectrum (red line).
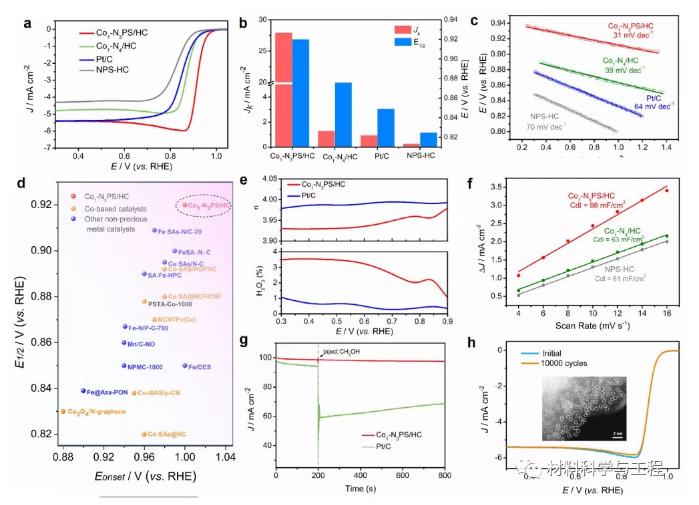
Figure 4. Electrocatalytic alkaline ORR performance of Co1-N3PS/HC. (A) Schematic diagram of the ORR polarization curve of Co1-N3PS/HC and reference catalyst in 0.1 M KOH. (B) Compare the Jk and E1/2 of Co1-N3PS/HC and the reference catalyst at 0.90 V. (C) Tafel diagram of Co1-N3PS/HC and reference catalyst. (D) Comparison of Co1-N3PS/HC in Table S4 with the previously reported Eonset and E1/2 of Co-based catalysts and other non-noble metal catalysts. (E) Schematic diagram of the electron transfer number of Co1-N3PS/HC (top) and H2O2 yield. (F) Electrochemical double layer capacitance (Cdl) of Co1-N3PS/HC and reference catalyst. (G) The tolerance curve of Co1N3PS/HC to methanol and the tolerance of 20% Pt/C at 0.70 V (inject methanol into 0.1 M KOH solution in 200 s). (H) The ORR polarization curves of Co1-N3PS/HC before and after 10,000 potential cycles (the inset is the AC HAADF-STEM image of Co1-N3PS/HC after durability test).
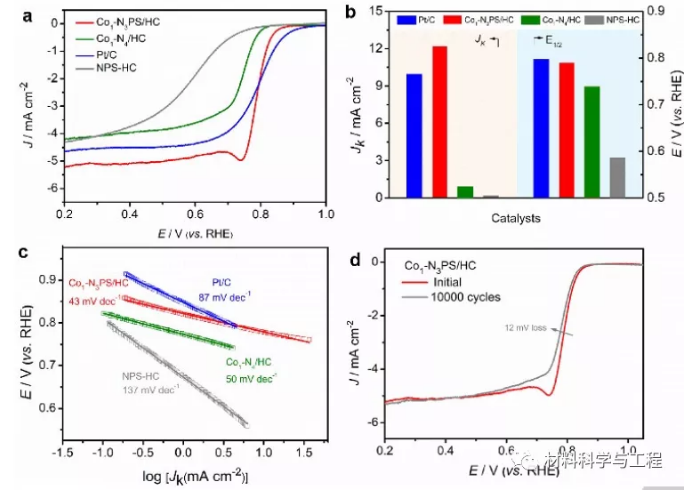
Figure 5. Electrocatalytic acid ORR performance of Co1-N3PS/HC. (A) Schematic diagram of ORR polarization curve of Co1-N3PS/HC and reference catalyst in 0.5 M H2SO4. (B) Comparison of the Jk and E1/2 schematic diagrams of Co1-N3PS/HC and the reference catalyst at 0.775 V. (C) Tafel diagram of Co1-N3PS/HC and reference catalyst. (D) Schematic diagram of the ORR polarization curve before and after 10,000 potential cycles of Co 1-N3PS/HC in 0.5 M H2SO4.
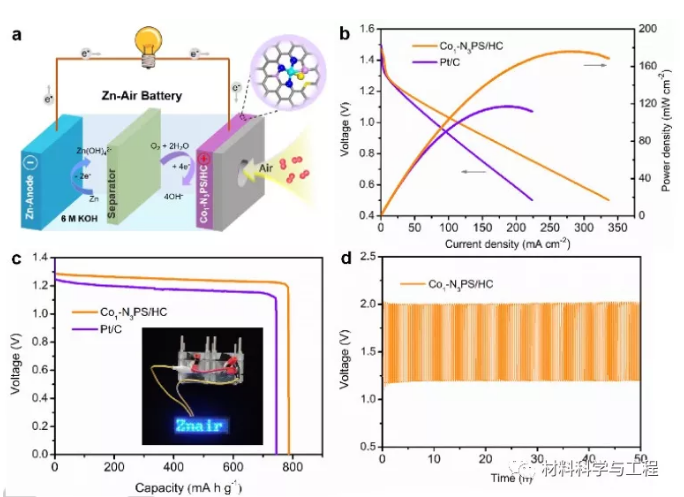
Figure 6. Co1-N3PS / HC zinc-air battery performance. (A) Schematic diagram of zinc-air battery. (B) The discharge polarization curve and power density diagram of zinc-air battery based on Co1-N3PS/HC and Pt/C. (C) The specific discharge capacity curve of Co1-N3PS/HC and Pt/C zinc-air batteries at 10 mA cm-2. (D) Constant current charge-discharge cycle curve of Co1-N3PS/HC-based zinc-air battery.
This paper proves that the ORR performance of the Co single-atom catalyst can be adjusted and optimized by accurately controlling the atomic configuration of the active site based on DFT calculation and electrochemical analysis. Guided by DFT calculations, a two-step pyrolysis strategy was developed to prepare a single-atom Co site catalyst with optimized CoN3PS active part. The atomic configuration of Co1-N3PS/HC has been confirmed by a series of advanced characterization techniques, including XPS analysis, AC HAADF-STEM and XAFS measurement. Co1-N3PS/HC shows high efficiency ORR reactivity and excellent ORR kinetics in both alkaline and acidic media, as well as good methanol tolerance and excellent stability, which is better than the commercially available Pt/C and large Most known non-noble metal electrocatalysts.
In addition, the zinc-air battery based on Co1N3PS/HC has excellent battery performance and long-term charge/discharge durability, proving its huge potential application prospects. According to the designed control experiment and DFT calculation, this paper confirms that the optimal electron density of the atomically dispersed Co active center caused by the unique coordinated coordination of N, P and S atoms plays a key role in the significant improvement of ORR performance. This work has improved the scientific understanding of the relationship between atomic site electron density and ORR performance, which will provide a new method for rational design and optimization of catalysts. (Text: SSC)
- Previous: In recent years, what
- Next: A Rising 2D Star: Nove