Dong Changming of Shanghai Jiaotong University: adjustable adhesion / micropore / bionic / glycopeptide hydrogel for rapid hemostasis and efficient wound healing
QQ Academic Group: 1092348845
Detailed
[abstract].
Although the first generation of tissue adhesives and hemostatic agents have been used in clinic, the microstructure of hydrogels and the correlation between hemostasis and wound healing are not clear. and it is difficult to design high-performance hydrogels to meet the growing global demand for wound closure, hemostasis and healing. Inspired by the microstructure of extracellular matrix and simulated chemistry of mussels, Professor Dong Changming, Jiayu Lu/ of Shanghai Jiaotong University, prepared two kinds of coordination and covalent glycopeptide hydrogels, which have adjustable tissue adhesion strength (14.6-83.9 kPa) and microporous structure (8-18 ¦Ì m), and the hemolysis rate is less than 1.5%.
It is worth noting that the micropore size mainly controls hemostasis. Compared with fibrin glue, the hydrogel with a pore size of 16-18 ¦Ì m has the fastest hemostatic speed, about 14 seconds, and the lowest blood loss, about 6%. In addition, biocompatibility and hemostasis affect wound healing, as assessed by hemolysis, cytotoxicity, subcutaneous implantation, and hemostasis and healing tests. Importantly, the rat skin defect model treated with glycopeptide hydrogel completely closed the wound and regenerated thick dermis and epidermis with some hair follicles on the 14th day.Therefore, this work not only establishes a general method for the construction of glycopeptide hydrogels with adjustable adhesion and microporous structure, rapid hemostasis and excellent healing function, but also reveals the useful principle of designing high-performance hemostatic and healing hydrogels. The related paper is published in Advanced Functional Materials under the title Biomimetic Glycopolypeptide Hydrogels with Tunable Adhesion and Microporous Structure for Fast Hemostasis and Highly Efficient Wound Healing.
[guide to the main picture].
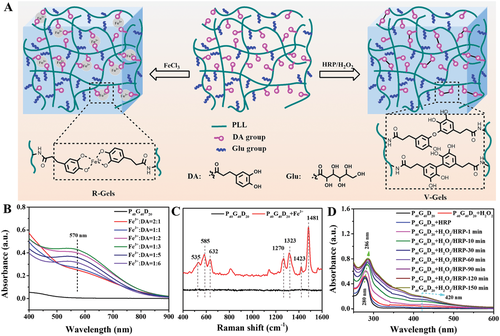
Fig. 1 preparation and characterization of glycopeptide hydrogel. A) schematic diagram of coordinated and covalent glycopeptide hydrogels (i.e. R-Gels and V-Gels). B) the UV-vis spectra of P40G40D20 in different amounts of FeCl3. C) Raman spectra of P40G40D20 with / without FeCl3. D) the UV-vis spectrum of P40G40D20 in HRP/H2O2 solution with time.
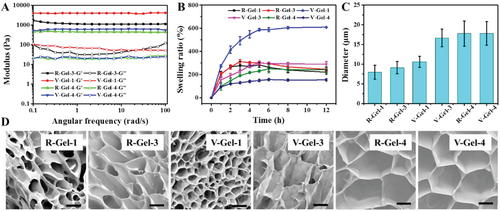
Fig. 2 physical and chemical properties and microstructure of hydrogels. A) rheology and B) swelling properties of hydrogel in PBS at 37 ¡ãC, C) pore diameter and D) morphology of freeze-dried gel (scale = 10 ¦Ì m).
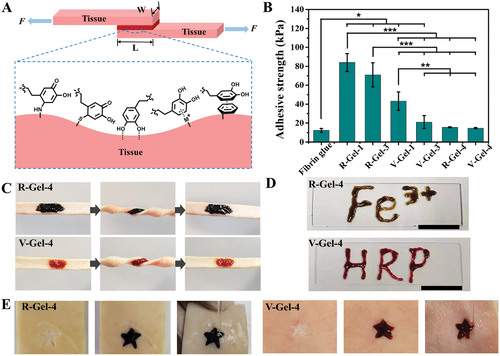
Fig. 3 Wet tissue adhesion and injectability of glycopeptide hydrogel. A) the schematic diagram of wet tissue adhesion test and the interface interaction between hydrogel and tissue. B) the adhesion strength of hydrogel to pigskin. C) A photo of a hydrogel adhering to pig skin under twisted conditions. D) A letter written by injecting hydrogel. E) even under the condition of water scintillation, the hydrogel can completely fill the irregular star-shaped wound.
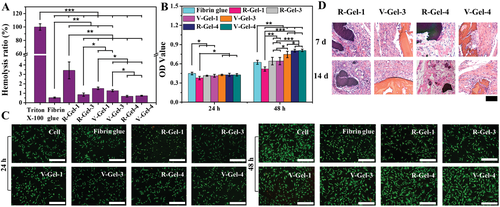
Fig. 4 biocompatibility of these glycopeptide hydrogels in vitro and in vivo. A) in vitro blood compatibility and B) cytocompatibility of hydrogel in vitro, C) double staining fluorescence images of rabbit living / dead cells incubating skin fibroblasts on the surface of hydrogel for 24 and 48 hours, and D) histocompatibility of hydrogel in vivo after subcutaneous implantation.
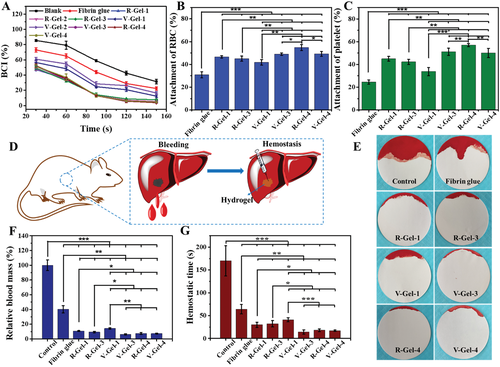
Fig. 5 Coagulation and hemostatic properties of these glycopeptide hydrogels. A) time dependence of coagulation index (BCI), and B) adhesion of red blood cells and C) platelets to the hydrogel. D) the diagram of the rat liver hemorrhage model, E) the bleeding condition, F) the relative blood loss, and G) the hemostasis time of the rat liver hemorrhage model treated with different hydrogels.
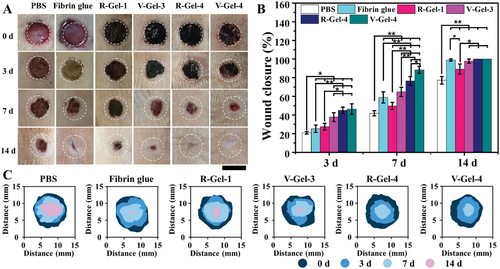
Fig. 6 full-thickness rat skin defect model for evaluating wound healing. A) representative wound photos, B) wound closure level, and C) wound area map of time evolution in each group.
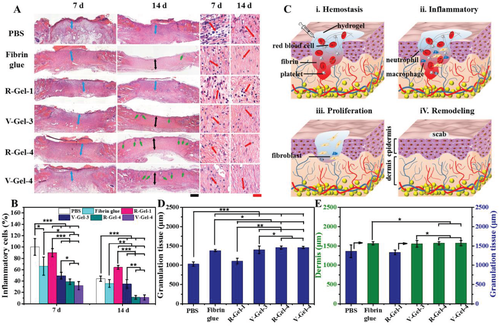
Figure 7A) histological images of Helie staining at low and high magnification (blue arrow: granulation tissue, black arrow: dermis, green arrow: hair follicles, red arrow: inflammatory cells, black scale = 500 ¦Ì m, red scale = 50 ¦Ì m). B) inflammatory cells in each group on the 7th and 14th day. C) A chart showing the four main stages of the wound healing process. D) thickness of granulation tissue on day 7 and E) thickness of dermis on day 14.
[summary].
The team prepared two kinds of coordinated and covalent glycopeptide hydrogels in a simple way, which have adjustable tissue adhesion strength, microporous structure, high water absorption and swelling, good injectability and irregular shape filling practicality. and low hemolysis. Whether covalent cross-linking or coordination cross-linking, those hydrogels with a pore size of 16-18 ¦Ì m achieved excellent hemostatic effect, with a minimum blood loss of about 6%, indicating that micropore size is a key factor in controlling hemostasis in vivo. In addition, both biocompatibility and hemostasis affect the wound healing properties of hydrogels and the complete wound healing of thick dermis, epidermis and some hair follicles on a 14-day full-thickness rat skin defect model. It is worth noting that this work reveals the correlation between microstructure and hemostasis in vivo and wound healing in hydrogels, and provides a useful basic principle for the design of hemostatic and healing adhesives and dressings based on high-performance hydrogels.
This information is from the Internet for academic exchange only. if there is any infringement, please contact us to delete it immediately.
Although the first generation of tissue adhesives and hemostatic agents have been used in clinic, the microstructure of hydrogels and the correlation between hemostasis and wound healing are not clear. and it is difficult to design high-performance hydrogels to meet the growing global demand for wound closure, hemostasis and healing. Inspired by the microstructure of extracellular matrix and simulated chemistry of mussels, Professor Dong Changming, Jiayu Lu/ of Shanghai Jiaotong University, prepared two kinds of coordination and covalent glycopeptide hydrogels, which have adjustable tissue adhesion strength (14.6-83.9 kPa) and microporous structure (8-18 ¦Ì m), and the hemolysis rate is less than 1.5%.
It is worth noting that the micropore size mainly controls hemostasis. Compared with fibrin glue, the hydrogel with a pore size of 16-18 ¦Ì m has the fastest hemostatic speed, about 14 seconds, and the lowest blood loss, about 6%. In addition, biocompatibility and hemostasis affect wound healing, as assessed by hemolysis, cytotoxicity, subcutaneous implantation, and hemostasis and healing tests. Importantly, the rat skin defect model treated with glycopeptide hydrogel completely closed the wound and regenerated thick dermis and epidermis with some hair follicles on the 14th day.Therefore, this work not only establishes a general method for the construction of glycopeptide hydrogels with adjustable adhesion and microporous structure, rapid hemostasis and excellent healing function, but also reveals the useful principle of designing high-performance hemostatic and healing hydrogels. The related paper is published in Advanced Functional Materials under the title Biomimetic Glycopolypeptide Hydrogels with Tunable Adhesion and Microporous Structure for Fast Hemostasis and Highly Efficient Wound Healing.
[guide to the main picture].
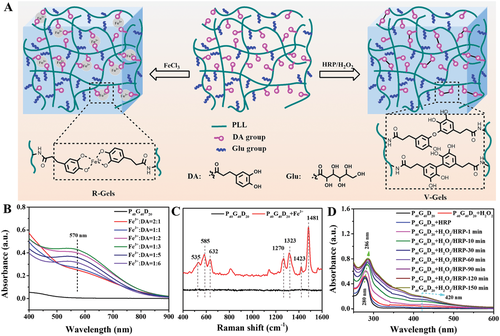
Fig. 1 preparation and characterization of glycopeptide hydrogel. A) schematic diagram of coordinated and covalent glycopeptide hydrogels (i.e. R-Gels and V-Gels). B) the UV-vis spectra of P40G40D20 in different amounts of FeCl3. C) Raman spectra of P40G40D20 with / without FeCl3. D) the UV-vis spectrum of P40G40D20 in HRP/H2O2 solution with time.
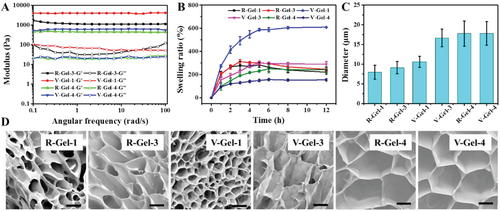
Fig. 2 physical and chemical properties and microstructure of hydrogels. A) rheology and B) swelling properties of hydrogel in PBS at 37 ¡ãC, C) pore diameter and D) morphology of freeze-dried gel (scale = 10 ¦Ì m).
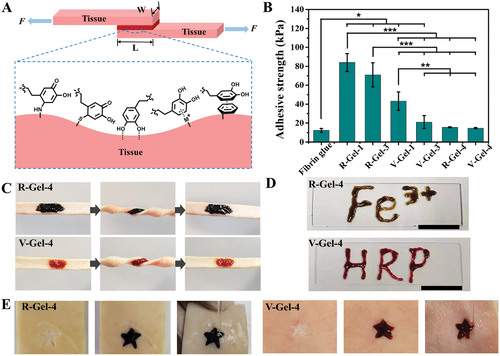
Fig. 3 Wet tissue adhesion and injectability of glycopeptide hydrogel. A) the schematic diagram of wet tissue adhesion test and the interface interaction between hydrogel and tissue. B) the adhesion strength of hydrogel to pigskin. C) A photo of a hydrogel adhering to pig skin under twisted conditions. D) A letter written by injecting hydrogel. E) even under the condition of water scintillation, the hydrogel can completely fill the irregular star-shaped wound.
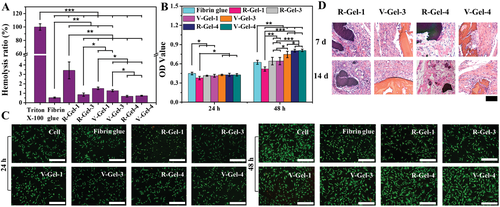
Fig. 4 biocompatibility of these glycopeptide hydrogels in vitro and in vivo. A) in vitro blood compatibility and B) cytocompatibility of hydrogel in vitro, C) double staining fluorescence images of rabbit living / dead cells incubating skin fibroblasts on the surface of hydrogel for 24 and 48 hours, and D) histocompatibility of hydrogel in vivo after subcutaneous implantation.
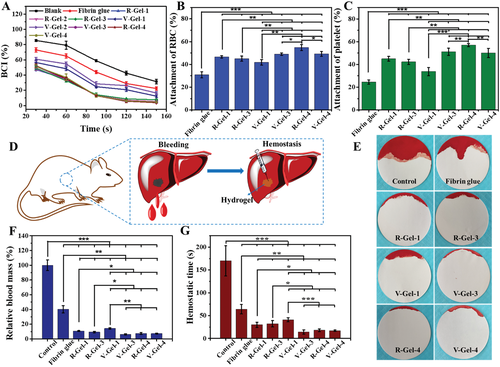
Fig. 5 Coagulation and hemostatic properties of these glycopeptide hydrogels. A) time dependence of coagulation index (BCI), and B) adhesion of red blood cells and C) platelets to the hydrogel. D) the diagram of the rat liver hemorrhage model, E) the bleeding condition, F) the relative blood loss, and G) the hemostasis time of the rat liver hemorrhage model treated with different hydrogels.
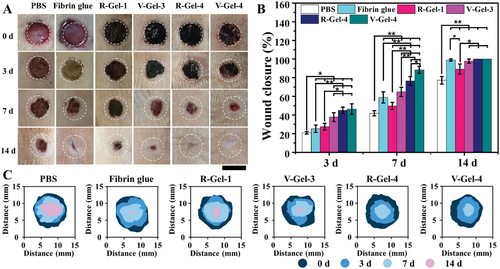
Fig. 6 full-thickness rat skin defect model for evaluating wound healing. A) representative wound photos, B) wound closure level, and C) wound area map of time evolution in each group.
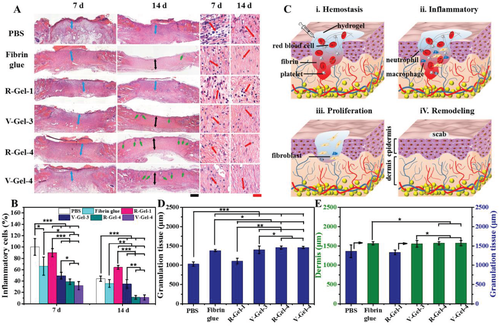
Figure 7A) histological images of Helie staining at low and high magnification (blue arrow: granulation tissue, black arrow: dermis, green arrow: hair follicles, red arrow: inflammatory cells, black scale = 500 ¦Ì m, red scale = 50 ¦Ì m). B) inflammatory cells in each group on the 7th and 14th day. C) A chart showing the four main stages of the wound healing process. D) thickness of granulation tissue on day 7 and E) thickness of dermis on day 14.
[summary].
The team prepared two kinds of coordinated and covalent glycopeptide hydrogels in a simple way, which have adjustable tissue adhesion strength, microporous structure, high water absorption and swelling, good injectability and irregular shape filling practicality. and low hemolysis. Whether covalent cross-linking or coordination cross-linking, those hydrogels with a pore size of 16-18 ¦Ì m achieved excellent hemostatic effect, with a minimum blood loss of about 6%, indicating that micropore size is a key factor in controlling hemostasis in vivo. In addition, both biocompatibility and hemostasis affect the wound healing properties of hydrogels and the complete wound healing of thick dermis, epidermis and some hair follicles on a 14-day full-thickness rat skin defect model. It is worth noting that this work reveals the correlation between microstructure and hemostasis in vivo and wound healing in hydrogels, and provides a useful basic principle for the design of hemostatic and healing adhesives and dressings based on high-performance hydrogels.
This information is from the Internet for academic exchange only. if there is any infringement, please contact us to delete it immediately.
- Previous£º Gold nanoclusters with
- Next£º A Rising 2D Star: Nove