Seed-like MXene-based composites: enhanced microwave absorption by polarization and magnetization
QQ Academic Group: 1092348845
Detailed
BeiKonami can provide "seed-like" MXene-based composites (customizable)
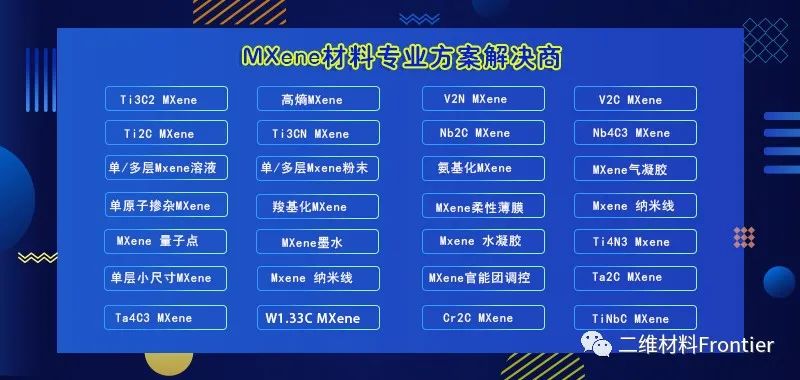
Theoretically, a microwave absorbing material with excellent performance must first meet the appropriate impedance matching conditions (Z value) and good attenuation coefficient (α value), which are jointly determined by its microscopic conductivity loss, polarization loss and magnetic loss. of. A suitable Z value means that more incident electromagnetic waves can enter the material system, thereby suppressing the reflection behavior of incident electromagnetic waves on the material surface, which is also a prerequisite for further attenuation and dissipation of electromagnetic waves. Excessive conductance loss will directly result in mismatched Z values, resulting in materials exhibiting poor absorbing properties. The good conductivity loss, polarization loss and magnetic loss ability make the material have high α value, showing excellent electromagnetic wave loss ability. From this point of view, there is a certain contradiction between improving the Z value and increasing the α value at the same time. Therefore, the principle of designing the absorbing system should take into account the Z value and the α value at the same time, and the polarization loss and magnetic loss ability of the material should be improved as much as possible in the microscopic mechanism, and the electrical conductivity should be maintained at an appropriate level.
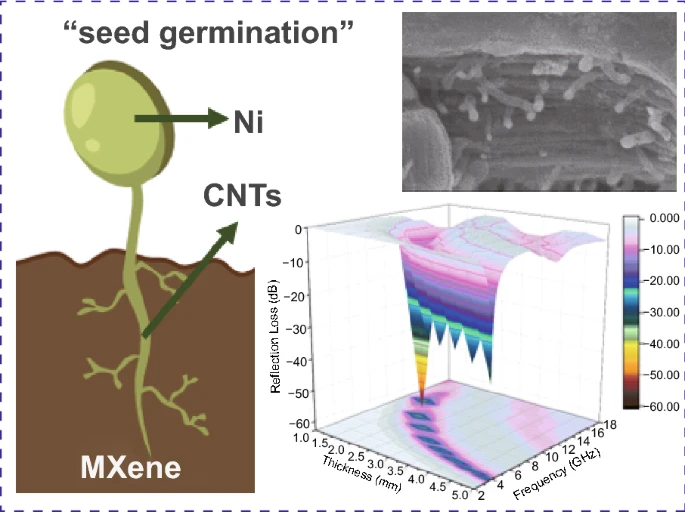
3D Seed-Germination-Like MXene with In Situ Growing CNTs/Ni Heterojunction for Enhanced Microwave Absorption via Polarization and Magnetization
Xiao Li, Wenbin You, Chunyang Xu, Lei Wang, Liting Yang, Yuesheng Li, Renchao Che*
Nano-Micro Letters (2021) 13: 157
https://doi.org/10.1007/s40820-021-00680-w
Highlights of this article
1. The composite has a typical "seed germination"-like morphology structure: multilayer MXene acts as "soil", and Ni ions embedded in it play the role of "seeds" that break out of the ground, and then catalyze the growth to grow on "branchs". The "buds" (Ni particles) at the top of the stem" (CNTs).
2. Compared with the easy agglomeration phenomenon of conventional magnetic particles, the MXene-CNTs/Ni composites exhibit a highly dispersed spatial magnetic structure.
3. The MXene-CNTs/Ni composite exhibits excellent microwave absorption performance (-56.4 dB with a coating thickness of 2.4 mm).
brief introduction
Mxene material, as a "new star" in the field of 2D materials, its unique accordion-like multilayer structure provides a natural structural advantage for extending the dissipation path of incident electromagnetic waves. However, the low dielectric loss capability and the lack of magnetic loss capability severely limit the further improvement of its performance. Inspired by the magnetoelectric synergy, the research group of Che Renchao of Fudan University solved this problem by preparing a magnetic CNTs/Ni heterostructure-modified MXene material by a simple in-situ induction method. At the same time, using in situ electron microscopy and electron holography techniques, the microscopic polarization and magnetization behavior of the material were elucidated in depth.
Graphical guide
I Design of "seed-sprouted" MXene-CNTs/Ni composites based on the principle of magnetoelectric synergy
First, the parent material MAX was etched in HF acid to obtain an accordion-shaped multilayer MXene, and then immersed in LiOH solution for alkalization treatment to further expand the interlayer spacing. Secondly, nickel chloride hexahydrate and melamine (mass ratio 1:6) were added into it and mixed well, due to ion exchange and electrostatic interaction, Ni ions could be attached to the surface and layer of each independently existing multilayer accordion-shaped MXene unit between. During calcination under nitrogen atmosphere, Ni ions were reduced to magnetic Ni nanoparticles and acted as catalysts to catalyze the in situ growth of CNTs (Figure 1). According to the calculation of XRD images, the interlayer spacing of the MXene-alk sample after alkali treatment increased from 0.953 nm to 1.413 nm, indicating that the alkalization treatment effectively increased the interlayer spacing of MXene and provided sufficient space for interlayer filling. space. The average interlayer spacing of MXene-CNTs/Ni was slightly reduced to 1.243 nm because part of the CNTs/Ni was grown in the interlayer of the multilayer MXene.
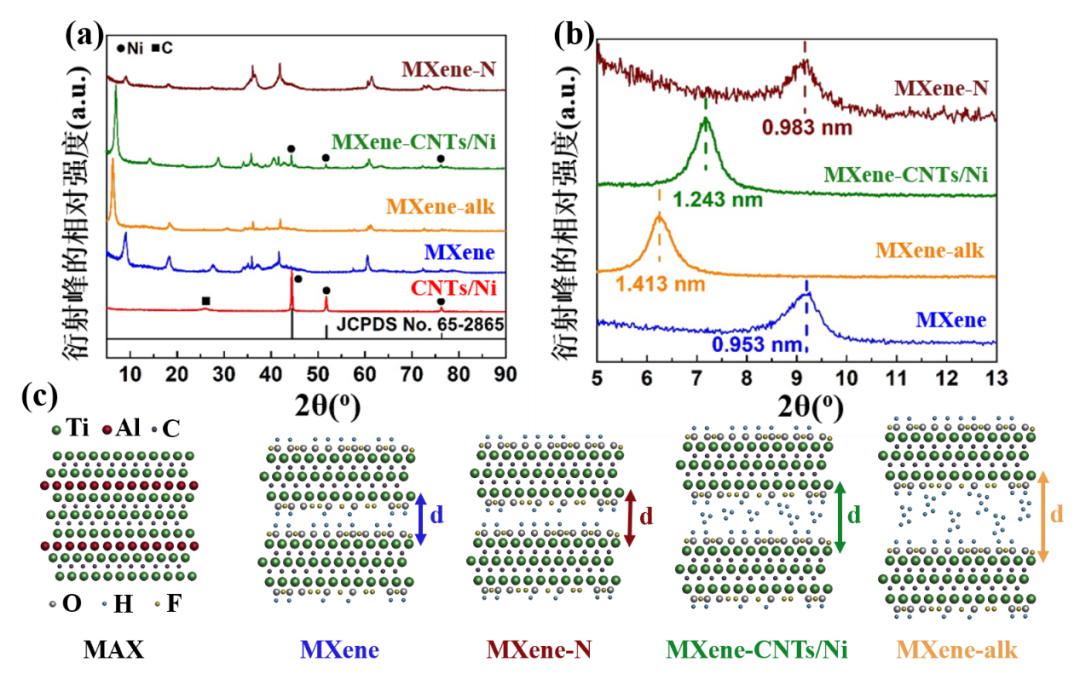
Figure 2. (a) XRD patterns of CNTs/Ni, MXene, MXene-alk, MXene-CNTs/Ni and MXene-N, (b) enlarged view of (a), (c) lattices of five different materials Model diagram.
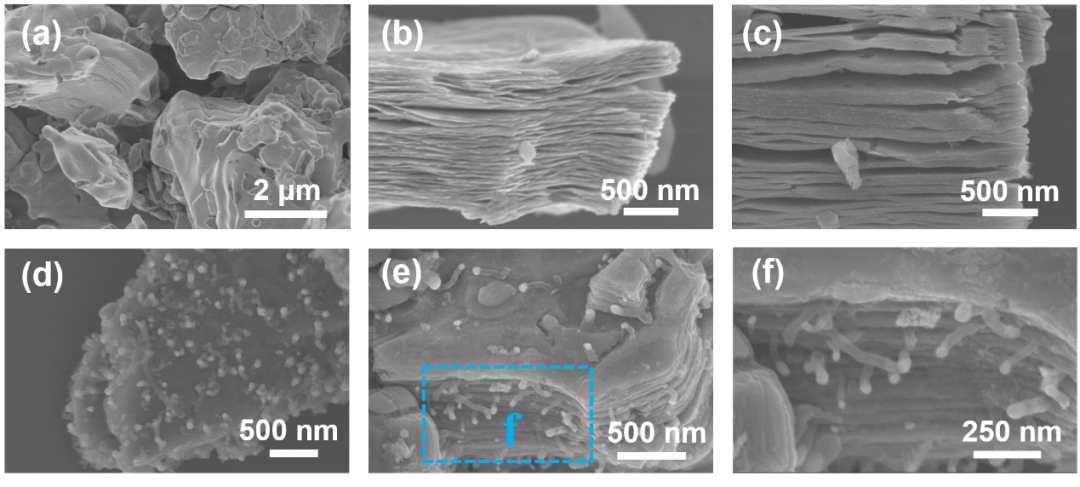
Figure 3. SEM images of (a) MAX, (b) MXene, (c) MXene-alk, (d-f) MXene-CNTs/Ni.
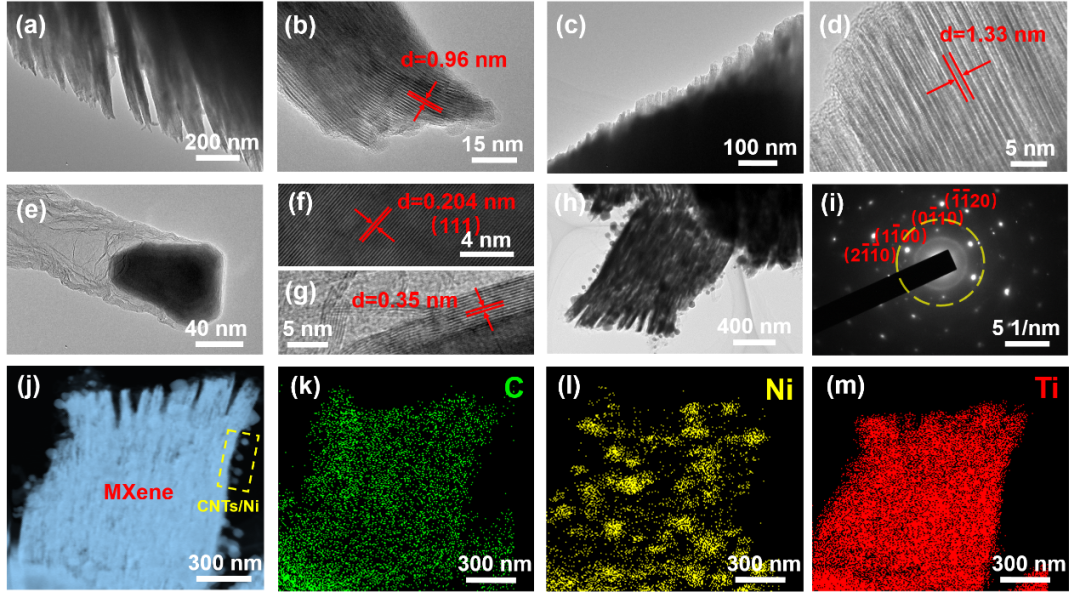
Figure 4. (a) TEM image and (b) HRTEM image of MXene, (c) TEM image and (d) HRTEM image of MXene-alk, (e) TEM image and (f, g) HRTEM image of CNTs/Ni , (h) TEM image and (i) SAED image of MXene-CNTs/Ni, (j) STEM image of MXene-CNTs/Ni and corresponding element distribution maps: (k) C, (l) Ni and (m ) Ti.
II Microwave absorption properties of "seed-like" MXene-CNTs/Ni composites
MXene and MXene-N exhibit poor wave absorbing properties due to insufficient dielectric loss capability and lack of magnetic loss capability. CNTs/Ni also shows poor absorbing performance, with the strongest reflection loss value of -22.3 dB at 5.2 GHz, which is due to the unbalanced impedance matching conditions caused by the high dielectric loss value. In contrast, MXene-CNTs/Ni exhibits excellent wave-absorbing properties, with a reflection loss value as high as -56.4 dB when the thickness is only 2.4 mm, and its effective absorption bandwidth is 3.95 GHz when the thickness is further adjusted to 1.5 mm (Fig. 5).
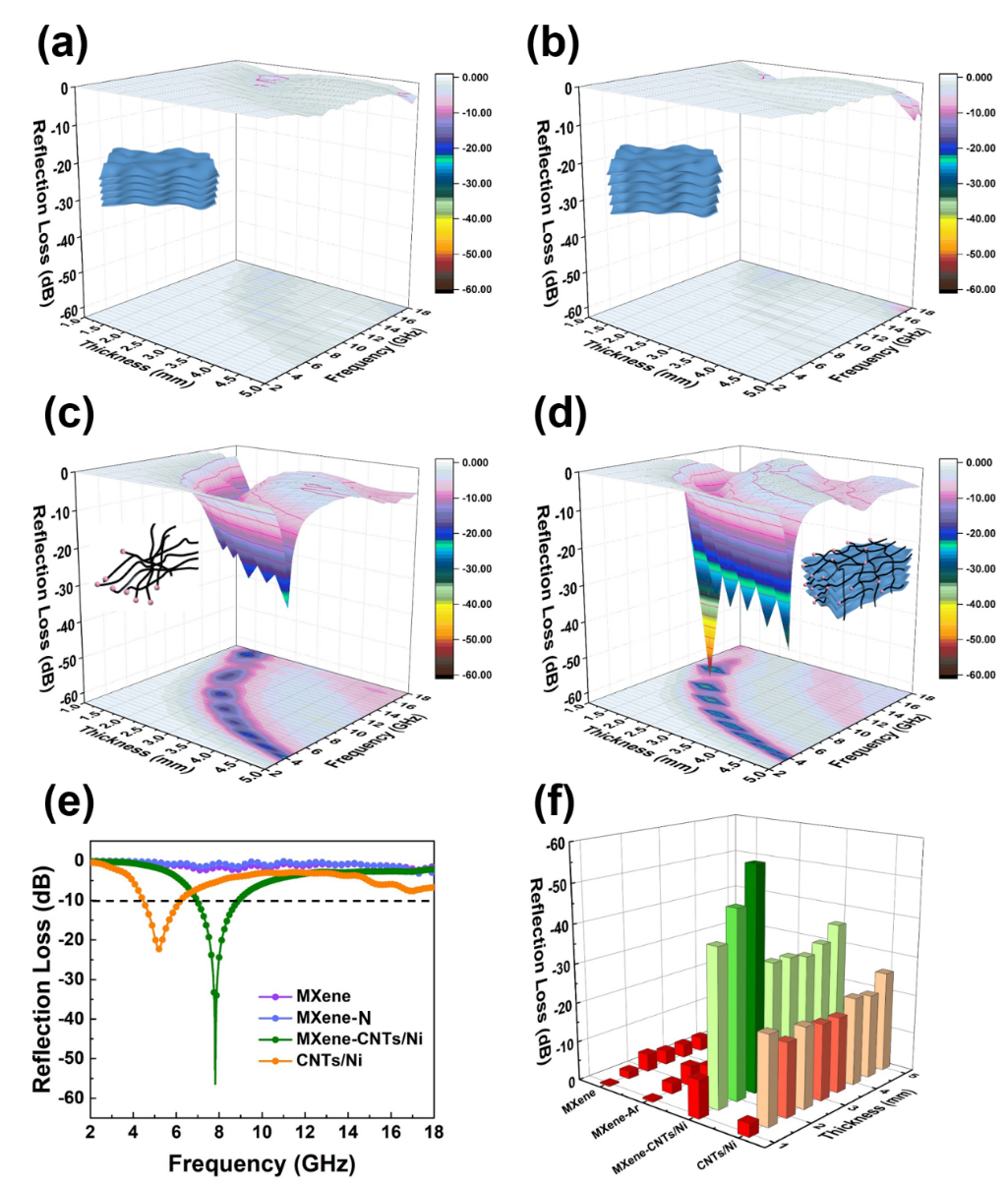
Figure 5. 3D plots of reflection loss values as a function of frequency and coating thickness for (a) MXene, (b) MXene-N, (c) CNTs/Ni and (d) MXene-CNTs/Ni, (e) four different A plot of the material at its respective strongest reflection loss value versus frequency, (f) a histogram of the reflection loss value versus frequency versus coating thickness for four different materials.
III Microscopic uptake mechanism of "seed-like" MXene-CNTs/Ni composites
The microscopic absorption mechanisms related to MXene-CNTs/Ni composites mainly include the following aspects:
i) Surrounding magnetic coupling network formed by highly dispersed spatial Ni particles. On the one hand, the bamboo-like CNTs with a large number of connection points can firmly support and separate the embedded magnetic Ni particles; Aggregation and size inhomogeneity issues. Electron holography results show that the embedded Ni particles show high-density magnetic lines of force and can penetrate the dielectric carbon wall and scatter to free space, confirming that the mixture exhibits magnetic outward and has the ability to magnetic loss. In the MXene-CNTs/Ni system, the high aspect ratio CNTs can "bridge" the non-magnetic MXene substrate and the magnetic Ni particles, so that the spatially highly dispersed magnetic particles surround each independently existing multilayer accordion-like interlayer. Electric unit, showing magnetism to the outside. The magnetic field lines between adjacent magnetic particles fuse with each other to form a magnetic coupling network on the micrometer scale, which exceeds the traditional magnetic coupling behavior based on the nanometer scale. The strong magnetic induction signal will strongly interact with the incident electromagnetic wave, thereby further enhancing the magnetic loss capability of the MXene-CNTs/Ni ternary composite system.
ii) Extensive heterointerfaces and defect sites between ternary complexes. The ability of magnetic loss mainly depends on the strength of magnetism, and the interface polarization and dipole polarization jointly contribute to the dielectric loss ability of the material. Compared with the binary system, the ternary complex has more heterogeneous interfaces in the phase composition. The area marked by the white arrow in Figure 7 is the upper wall of the carbon tube-Ni particles-the lower wall of the carbon tube, the corresponding charge density distribution colors are yellow-blue-yellow, representing different degrees of charge accumulation, and the corresponding charge types are Negative charge - positive charge - negative charge. Notably, a large amount of charges would accumulate at its interface, demonstrating the enhanced interface polarizability. Therefore, under the alternating electromagnetic field, a large number of carriers will rapidly migrate and accumulate at a large number of heterointerfaces, which together lead to the improvement of the interface polarizability. Ni particles will inevitably generate a large number of defects in the process of catalyzing the in-situ growth of carbon tubes. We use the geometric phase analysis technique to analyze the strain centers in the Ni particles, as shown in Figure 7f. The appearance of a large number of color inversion points indicates that There are many crystal defects in Ni particles. At the same time, the surface of MXene nanosheets will carry many functional groups and defect sites during the process of HF etching (Fig. 7h). These defect sites can be considered as dipole active sites. When electrons pass through these sites, they will This results in a strong dipole polarization behavior, which enables the ternary MXene-CNTs/Ni composite with significantly improved dielectric loss capability.
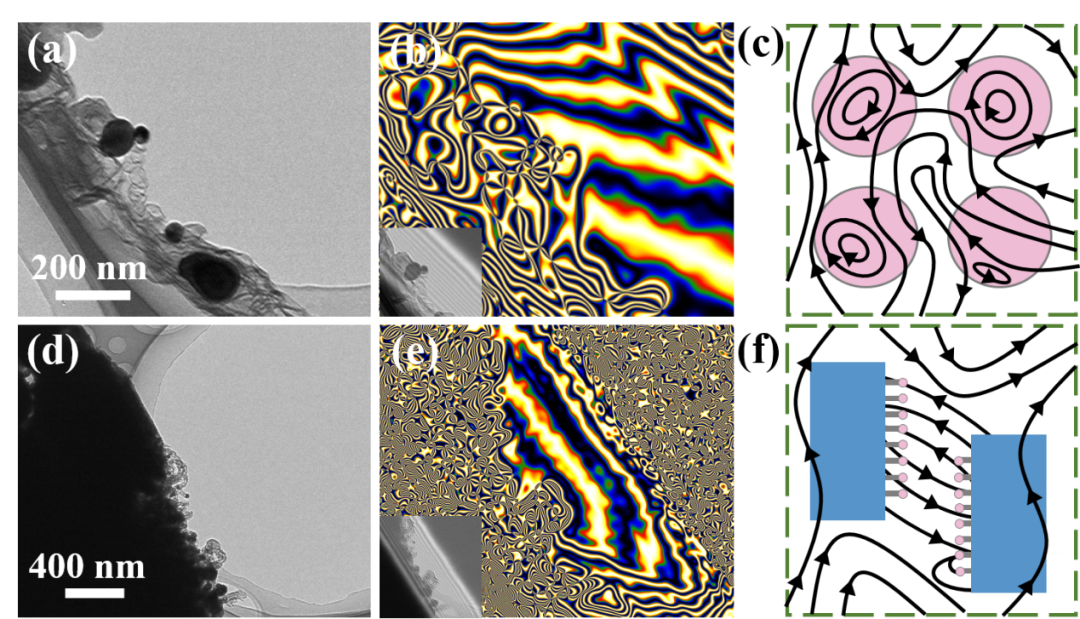
Figure 6. (a) TEM image of CNTs/Ni, (b) distribution of magnetic field lines and (c) schematic diagram of the corresponding magnetic coupling network, (d) TEM image of MXene-CNTs/Ni, (e) distribution of magnetic field lines and ( f) Schematic diagram of the corresponding magnetic coupling network.
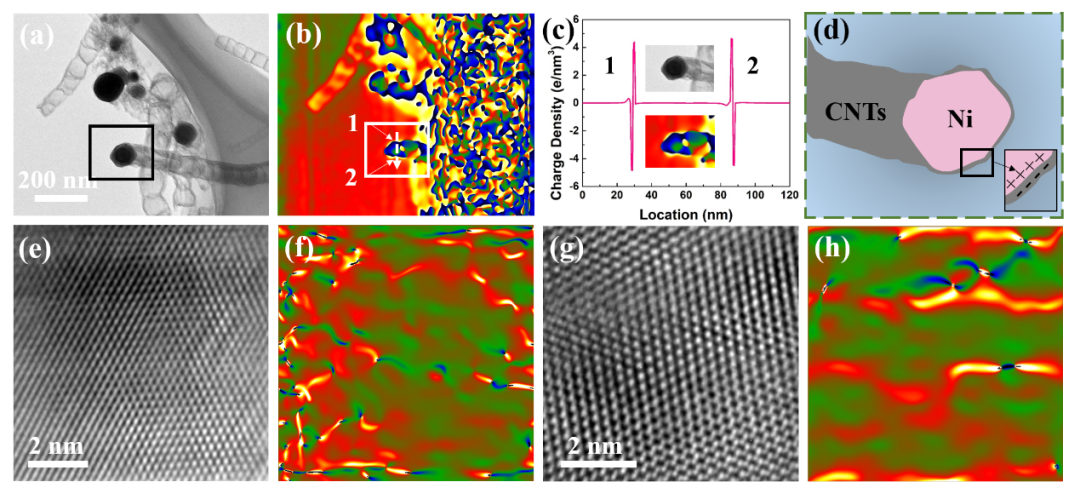
Figure 7. (a) TEM image of CNTs/Ni, (b) charge density distribution image, (c) charge density distribution curve image and (d) corresponding equivalent model image, (e) HRTEM image of Ni particles and ( f) Corresponding strain map, (g) HRTEM image and (h) corresponding strain map of MXene nanosheets. Color scale values are from -0.5 to +0.5 from black to white.
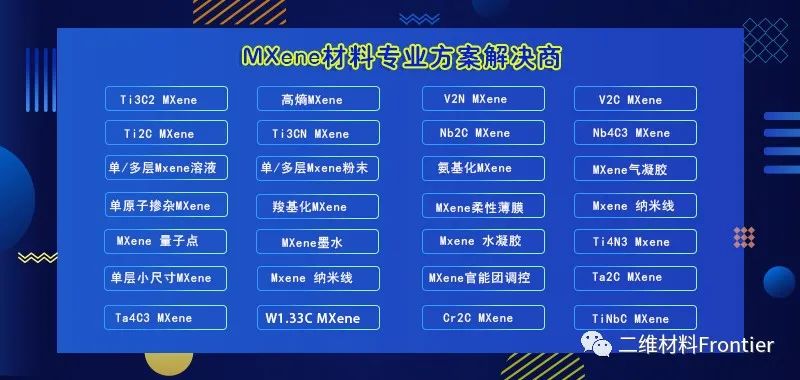
Theoretically, a microwave absorbing material with excellent performance must first meet the appropriate impedance matching conditions (Z value) and good attenuation coefficient (α value), which are jointly determined by its microscopic conductivity loss, polarization loss and magnetic loss. of. A suitable Z value means that more incident electromagnetic waves can enter the material system, thereby suppressing the reflection behavior of incident electromagnetic waves on the material surface, which is also a prerequisite for further attenuation and dissipation of electromagnetic waves. Excessive conductance loss will directly result in mismatched Z values, resulting in materials exhibiting poor absorbing properties. The good conductivity loss, polarization loss and magnetic loss ability make the material have high α value, showing excellent electromagnetic wave loss ability. From this point of view, there is a certain contradiction between improving the Z value and increasing the α value at the same time. Therefore, the principle of designing the absorbing system should take into account the Z value and the α value at the same time, and the polarization loss and magnetic loss ability of the material should be improved as much as possible in the microscopic mechanism, and the electrical conductivity should be maintained at an appropriate level.
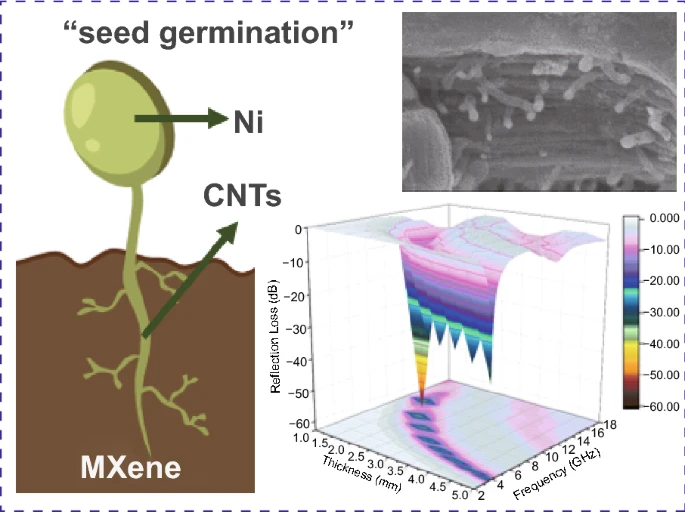
3D Seed-Germination-Like MXene with In Situ Growing CNTs/Ni Heterojunction for Enhanced Microwave Absorption via Polarization and Magnetization
Xiao Li, Wenbin You, Chunyang Xu, Lei Wang, Liting Yang, Yuesheng Li, Renchao Che*
Nano-Micro Letters (2021) 13: 157
https://doi.org/10.1007/s40820-021-00680-w
Highlights of this article
1. The composite has a typical "seed germination"-like morphology structure: multilayer MXene acts as "soil", and Ni ions embedded in it play the role of "seeds" that break out of the ground, and then catalyze the growth to grow on "branchs". The "buds" (Ni particles) at the top of the stem" (CNTs).
2. Compared with the easy agglomeration phenomenon of conventional magnetic particles, the MXene-CNTs/Ni composites exhibit a highly dispersed spatial magnetic structure.
3. The MXene-CNTs/Ni composite exhibits excellent microwave absorption performance (-56.4 dB with a coating thickness of 2.4 mm).
brief introduction
Mxene material, as a "new star" in the field of 2D materials, its unique accordion-like multilayer structure provides a natural structural advantage for extending the dissipation path of incident electromagnetic waves. However, the low dielectric loss capability and the lack of magnetic loss capability severely limit the further improvement of its performance. Inspired by the magnetoelectric synergy, the research group of Che Renchao of Fudan University solved this problem by preparing a magnetic CNTs/Ni heterostructure-modified MXene material by a simple in-situ induction method. At the same time, using in situ electron microscopy and electron holography techniques, the microscopic polarization and magnetization behavior of the material were elucidated in depth.
Graphical guide
I Design of "seed-sprouted" MXene-CNTs/Ni composites based on the principle of magnetoelectric synergy
First, the parent material MAX was etched in HF acid to obtain an accordion-shaped multilayer MXene, and then immersed in LiOH solution for alkalization treatment to further expand the interlayer spacing. Secondly, nickel chloride hexahydrate and melamine (mass ratio 1:6) were added into it and mixed well, due to ion exchange and electrostatic interaction, Ni ions could be attached to the surface and layer of each independently existing multilayer accordion-shaped MXene unit between. During calcination under nitrogen atmosphere, Ni ions were reduced to magnetic Ni nanoparticles and acted as catalysts to catalyze the in situ growth of CNTs (Figure 1). According to the calculation of XRD images, the interlayer spacing of the MXene-alk sample after alkali treatment increased from 0.953 nm to 1.413 nm, indicating that the alkalization treatment effectively increased the interlayer spacing of MXene and provided sufficient space for interlayer filling. space. The average interlayer spacing of MXene-CNTs/Ni was slightly reduced to 1.243 nm because part of the CNTs/Ni was grown in the interlayer of the multilayer MXene.
Figure 1. Schematic diagram of the synthesis process of MXene-CNTs/Ni composites.
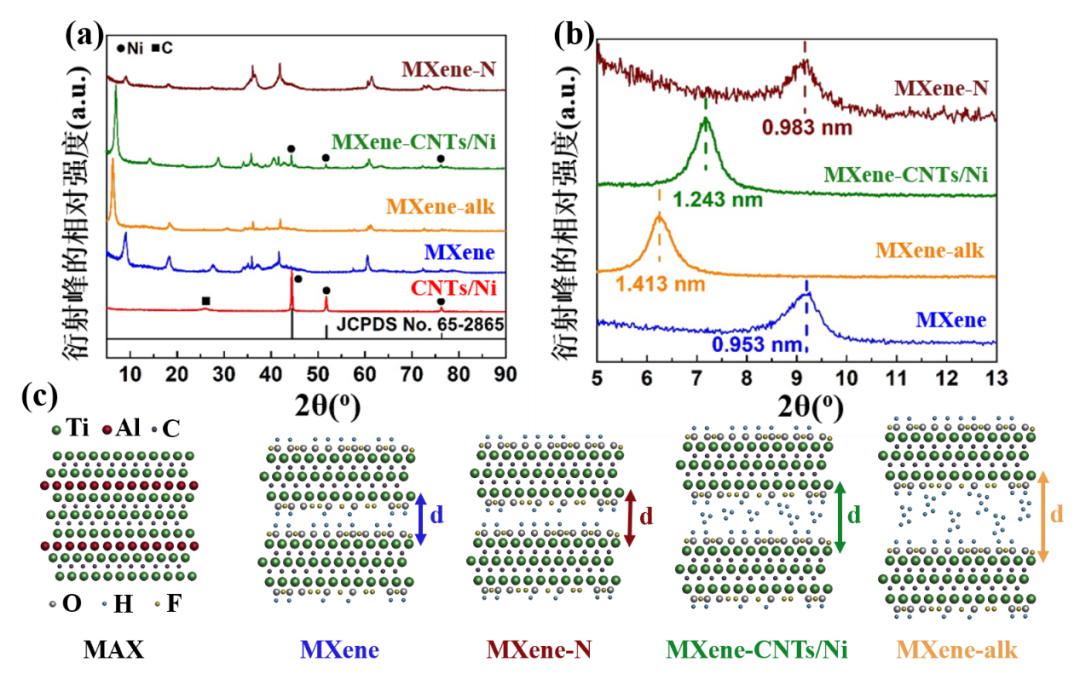
Figure 2. (a) XRD patterns of CNTs/Ni, MXene, MXene-alk, MXene-CNTs/Ni and MXene-N, (b) enlarged view of (a), (c) lattices of five different materials Model diagram.
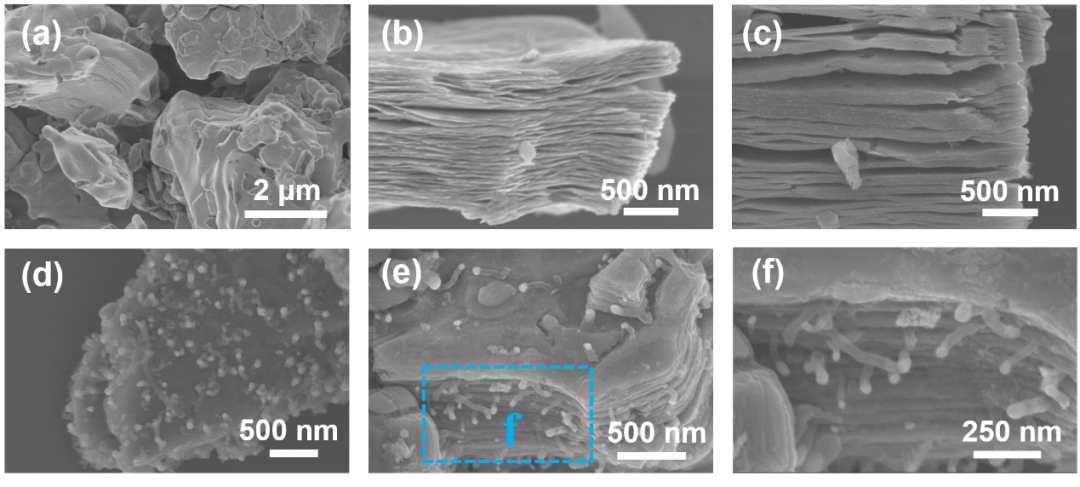
Figure 3. SEM images of (a) MAX, (b) MXene, (c) MXene-alk, (d-f) MXene-CNTs/Ni.
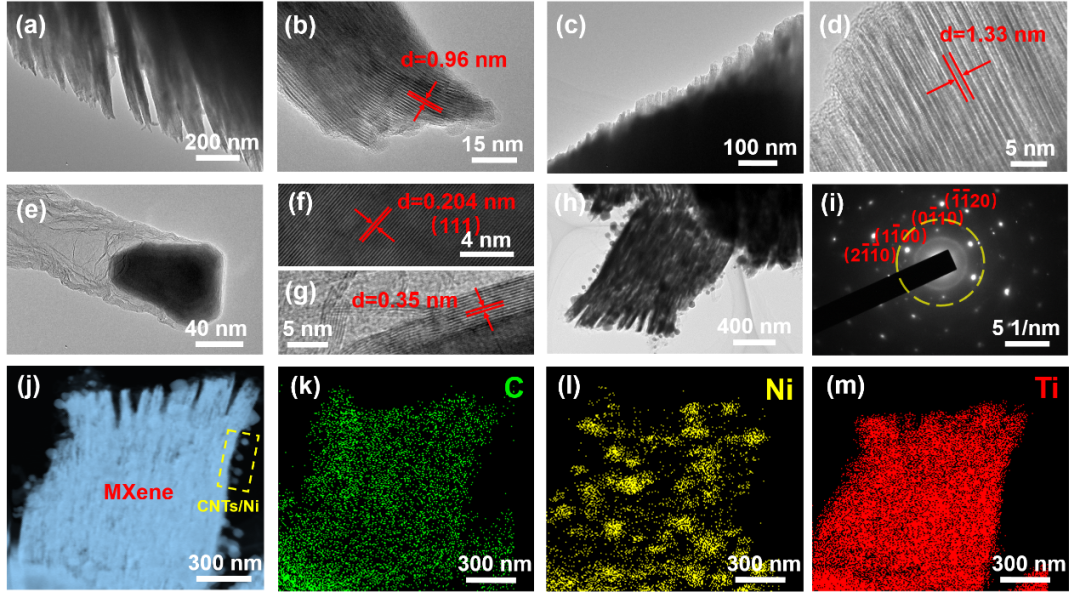
Figure 4. (a) TEM image and (b) HRTEM image of MXene, (c) TEM image and (d) HRTEM image of MXene-alk, (e) TEM image and (f, g) HRTEM image of CNTs/Ni , (h) TEM image and (i) SAED image of MXene-CNTs/Ni, (j) STEM image of MXene-CNTs/Ni and corresponding element distribution maps: (k) C, (l) Ni and (m ) Ti.
II Microwave absorption properties of "seed-like" MXene-CNTs/Ni composites
MXene and MXene-N exhibit poor wave absorbing properties due to insufficient dielectric loss capability and lack of magnetic loss capability. CNTs/Ni also shows poor absorbing performance, with the strongest reflection loss value of -22.3 dB at 5.2 GHz, which is due to the unbalanced impedance matching conditions caused by the high dielectric loss value. In contrast, MXene-CNTs/Ni exhibits excellent wave-absorbing properties, with a reflection loss value as high as -56.4 dB when the thickness is only 2.4 mm, and its effective absorption bandwidth is 3.95 GHz when the thickness is further adjusted to 1.5 mm (Fig. 5).
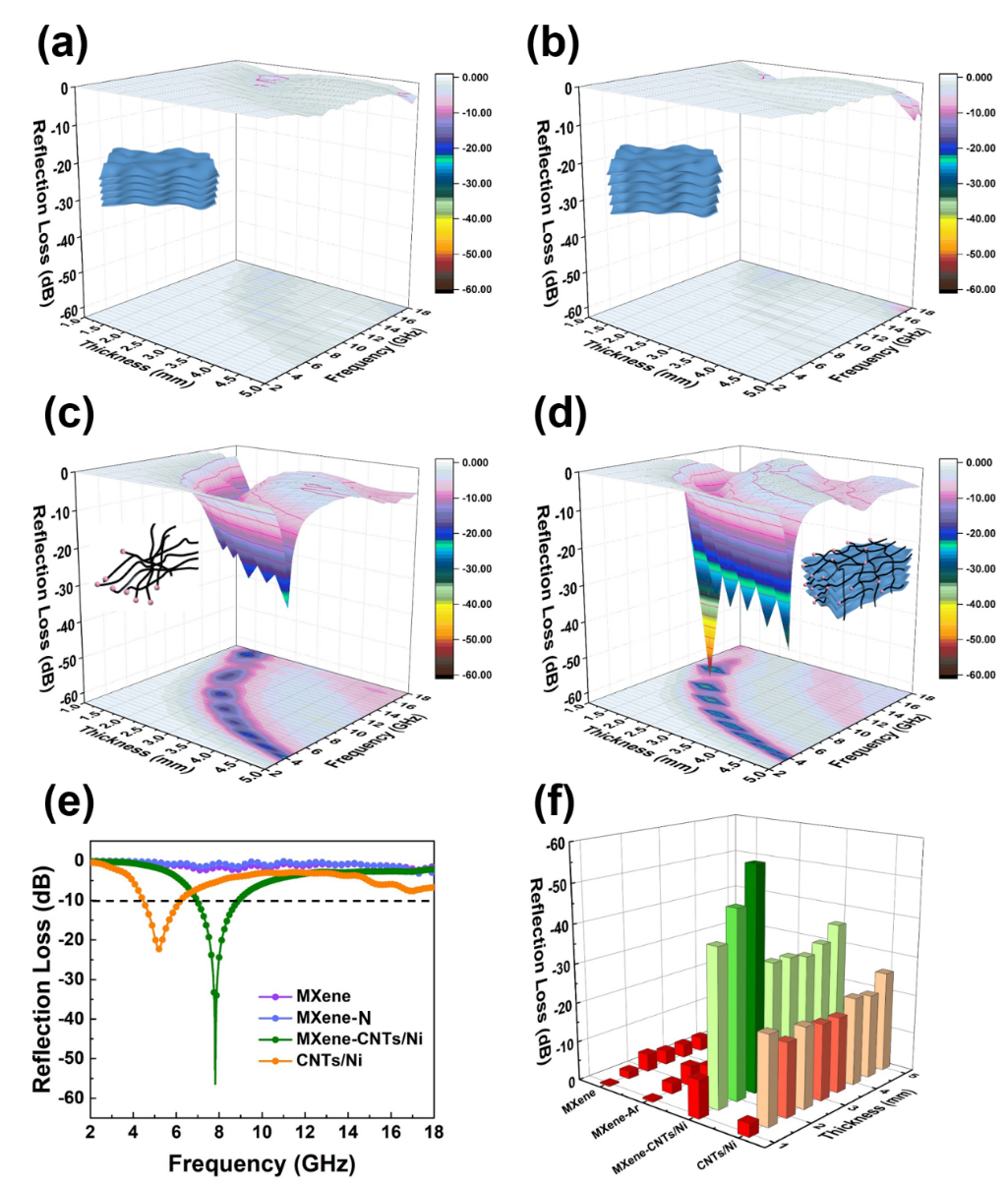
Figure 5. 3D plots of reflection loss values as a function of frequency and coating thickness for (a) MXene, (b) MXene-N, (c) CNTs/Ni and (d) MXene-CNTs/Ni, (e) four different A plot of the material at its respective strongest reflection loss value versus frequency, (f) a histogram of the reflection loss value versus frequency versus coating thickness for four different materials.
III Microscopic uptake mechanism of "seed-like" MXene-CNTs/Ni composites
The microscopic absorption mechanisms related to MXene-CNTs/Ni composites mainly include the following aspects:
i) Surrounding magnetic coupling network formed by highly dispersed spatial Ni particles. On the one hand, the bamboo-like CNTs with a large number of connection points can firmly support and separate the embedded magnetic Ni particles; Aggregation and size inhomogeneity issues. Electron holography results show that the embedded Ni particles show high-density magnetic lines of force and can penetrate the dielectric carbon wall and scatter to free space, confirming that the mixture exhibits magnetic outward and has the ability to magnetic loss. In the MXene-CNTs/Ni system, the high aspect ratio CNTs can "bridge" the non-magnetic MXene substrate and the magnetic Ni particles, so that the spatially highly dispersed magnetic particles surround each independently existing multilayer accordion-like interlayer. Electric unit, showing magnetism to the outside. The magnetic field lines between adjacent magnetic particles fuse with each other to form a magnetic coupling network on the micrometer scale, which exceeds the traditional magnetic coupling behavior based on the nanometer scale. The strong magnetic induction signal will strongly interact with the incident electromagnetic wave, thereby further enhancing the magnetic loss capability of the MXene-CNTs/Ni ternary composite system.
ii) Extensive heterointerfaces and defect sites between ternary complexes. The ability of magnetic loss mainly depends on the strength of magnetism, and the interface polarization and dipole polarization jointly contribute to the dielectric loss ability of the material. Compared with the binary system, the ternary complex has more heterogeneous interfaces in the phase composition. The area marked by the white arrow in Figure 7 is the upper wall of the carbon tube-Ni particles-the lower wall of the carbon tube, the corresponding charge density distribution colors are yellow-blue-yellow, representing different degrees of charge accumulation, and the corresponding charge types are Negative charge - positive charge - negative charge. Notably, a large amount of charges would accumulate at its interface, demonstrating the enhanced interface polarizability. Therefore, under the alternating electromagnetic field, a large number of carriers will rapidly migrate and accumulate at a large number of heterointerfaces, which together lead to the improvement of the interface polarizability. Ni particles will inevitably generate a large number of defects in the process of catalyzing the in-situ growth of carbon tubes. We use the geometric phase analysis technique to analyze the strain centers in the Ni particles, as shown in Figure 7f. The appearance of a large number of color inversion points indicates that There are many crystal defects in Ni particles. At the same time, the surface of MXene nanosheets will carry many functional groups and defect sites during the process of HF etching (Fig. 7h). These defect sites can be considered as dipole active sites. When electrons pass through these sites, they will This results in a strong dipole polarization behavior, which enables the ternary MXene-CNTs/Ni composite with significantly improved dielectric loss capability.
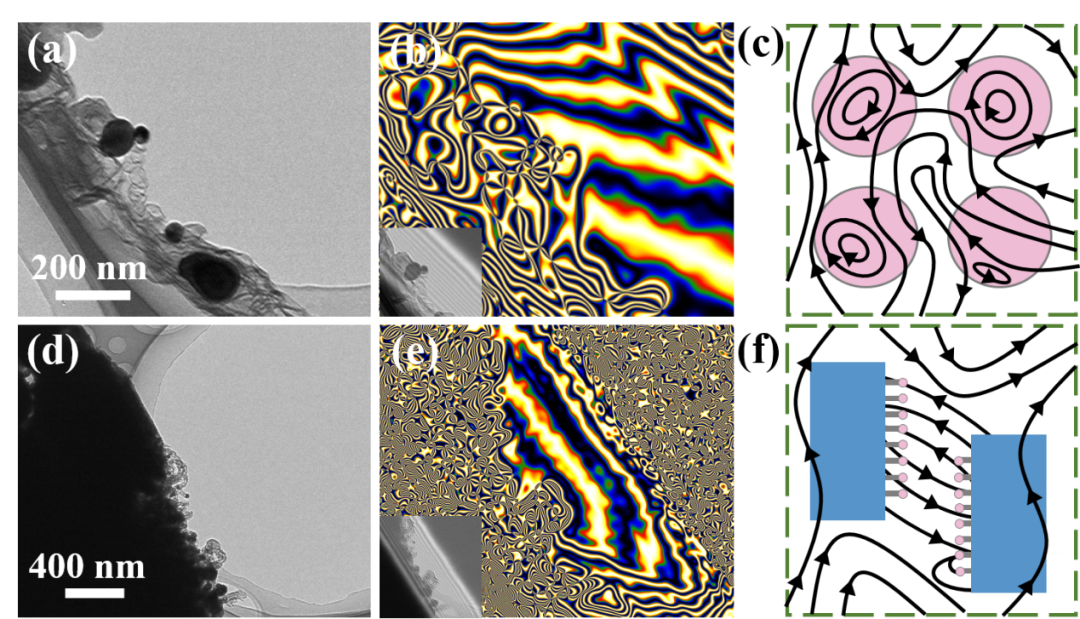
Figure 6. (a) TEM image of CNTs/Ni, (b) distribution of magnetic field lines and (c) schematic diagram of the corresponding magnetic coupling network, (d) TEM image of MXene-CNTs/Ni, (e) distribution of magnetic field lines and ( f) Schematic diagram of the corresponding magnetic coupling network.
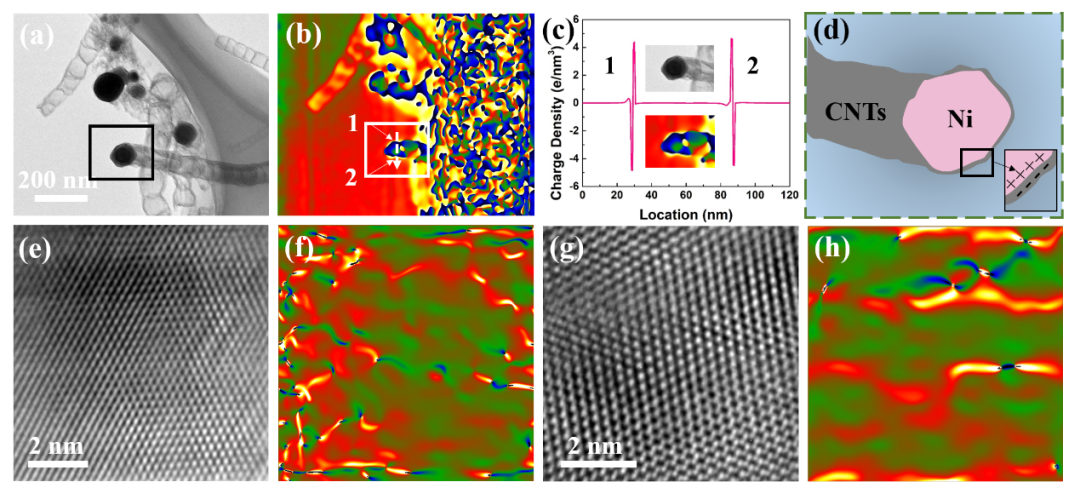
Figure 7. (a) TEM image of CNTs/Ni, (b) charge density distribution image, (c) charge density distribution curve image and (d) corresponding equivalent model image, (e) HRTEM image of Ni particles and ( f) Corresponding strain map, (g) HRTEM image and (h) corresponding strain map of MXene nanosheets. Color scale values are from -0.5 to +0.5 from black to white.
- Previous: J. Energy Chem.|Interf
- Next: MXene breakthrough: Na