Biointerface engineering of MXene nanosheets: photothermal enhancement of lysozyme activity for efficient sterilization of methicillin-resistant Staphylococcus aureus (MRSA)
QQ Academic Group: 1092348845
Detailed
MXene is a two-dimensional star material that is even hotter than graphene. As an advanced MXene manufacturer, North Konami offers special offers, 10% off mxene and other materials, and North Konami launches new mesoporous material products with more preferential prices , waiting for you to spike!
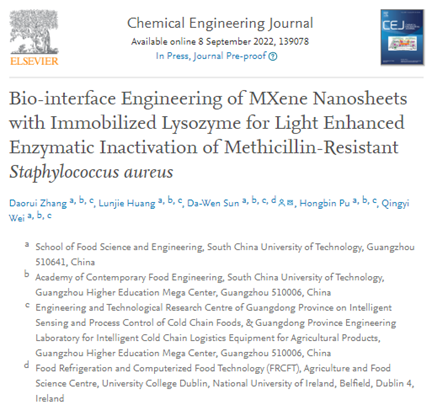
Research abstract
The emergence of drug-resistant bacteria has become a growing global public health problem. Among them, methicillin-resistant Staphylococcus aureus (MRSA) causes high morbidity and mortality. The existing antibacterial methods still have the problems of easily leading to new drug resistance, complex material synthesis, difficult purification and high cost. Therefore, there is an urgent need to develop a safe, efficient and low-cost antibacterial drug. Lysozyme in organisms can catalyze the hydrolysis of β-1,4-bonds between N-acetylmuramic acid and N-acetyl-D-glucosamine residues in peptidoglycan, thereby exhibiting antibacterial ability. Lysozyme can slow down the bacterial resistance caused by the overuse of antibiotics. However, as a biological macromolecule, the stability and antibacterial activity of lysozyme will be affected by the environment, and the immobilization method of enzyme emerges as the times require.
The new two-dimensional nanomaterial MXene has good biocompatibility and photothermal conversion ability, and is a good carrier for enzyme immobilization. Abundant surface functional groups (-OH, =O and -F) endow MXene with abundant anchoring sites. In addition, the excellent photothermal conversion performance of Ti3C2 MXene can realize photothermal sterilization, and under laser irradiation, the rapid increase of local temperature causes great damage to cell membranes and proteins, resulting in the death of bacteria. Compared with traditional antibacterial methods, photothermal antibacterial methods have the advantages of rapidity, high efficiency, non-invasiveness and environmental protection. In addition, studies in several fields have demonstrated the potential of MXenes in biointerface engineering and enzyme immobilization of biomolecules. However, previously published studies tend to combine MXenes with nanomaterials (such as noble metal nanoparticles) for antibacterial use, but the nanomaterials exhibit certain toxicity, which is not conducive to practical applications. In addition, there are no related reports on the immobilization of enzymes on MXene nanosheets for antibacterial studies.
Introduction
Recently, the research group of Professor Sun Dawen from the School of Food Science and Engineering of South China University of Technology has constructed a photothermal nano-antibacterial material based on MXene biointerface engineering, which can effectively kill MRSA by remotely regulating the activity of lysozyme by laser. Monolayer Ti3C2 MXene was functionalized by polydopamine (PDA) surface chemistry to enhance photothermal effect and stability, during which lysozyme was immobilized on this 2D hybrid interface by intermolecular electrostatic affinity. The photothermal conversion efficiency of the synthesized nanomaterials (denoted as M@P@Lyso) can reach 46.88%. While realizing the immobilization of lysozyme, the local temperature can be increased by laser regulation to activate the bactericidal activity of lysozyme. In vitro and in vivo antibacterial experiments showed that M@P@Lyso could effectively inhibit the proliferation of MRSA, accelerate wound healing in mice, and had excellent biocompatibility. The excellent antibacterial activity of M@P@Lyso should be attributed to the synergistic effect of multiple bactericidal mechanisms, i.e., the photothermal-promoted lysozyme biocatalytic activity, the local overheating effect generated by M@PDA, and the physical cleavage effect of the nanosheets. This study proposes a MXene-based method for enhancing the bactericidal effect of lysozyme by remote control of laser irradiation, which provides a technical reference for the development of highly stable and highly active antibacterial nanocomposites and their application.The result was published online in the top international journal Chemical Engineering Journal (impact factor 16.744) with the title: Bio-interface Engineering of MXene Nanosheets with Immobilized Lysozyme for Light Enhanced Enzymatic Inactivation of Methicillin-Resistant Staphylococcus aureus.
Graphical guide
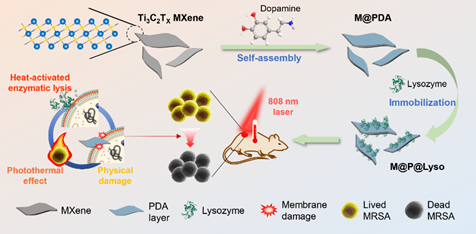
Figure 1. Schematic illustration of biointerface engineering of lysozyme on MXene and photothermal enhancement for sterilization of MRSA.
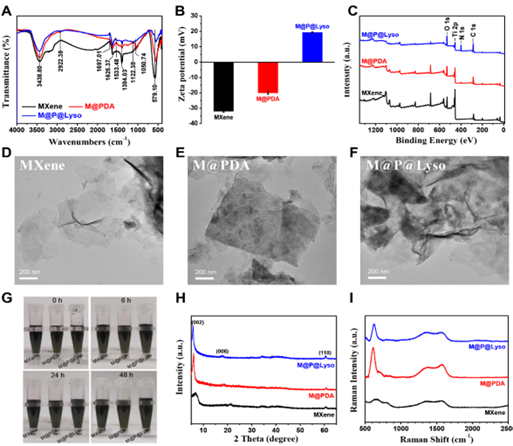
Figure 2. Characterization of synthesized nanomaterials. A) FTIR spectra, B) Zeta potential and C) XPS spectra of MXene, M@PDA and M@P@Lyso16. D) MXene, E) TEM images of M@PDA and F) M@P@Lyso. G) Photographs of the stability of MXene, M@PDA and M@P@Lyso16 in water at 100 μg/mL. H) XRD patterns and I) Raman spectra of MXene, M@P@Lyso4, M@P@Lyso8, M@P@Lyso12 and M@P@Lyso16.
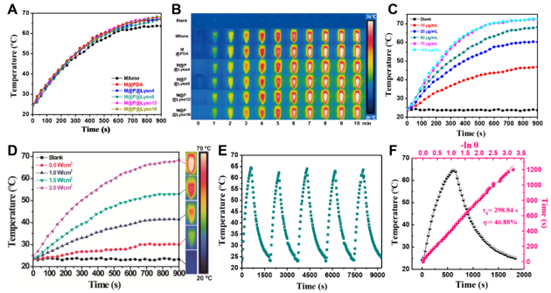
Figure 3. Electromagnetic shielding performance. a-c) Comparison of EMI SE, SET, SEA, and SER, and the electrical conductivity of MF-2, MSF-2, MCF-2, MCSF-10 with the same thickness of 40 μm. d, i) EMI SE, e) conductivity, f) image, h) comparison of SET, SEA and SER of MCSF-5, MCSF-10, MCSF-15, MCSF-20, MCSF-25, MCSF-30. j) Compare the characteristic EMI shielding effectiveness as a function of thickness.
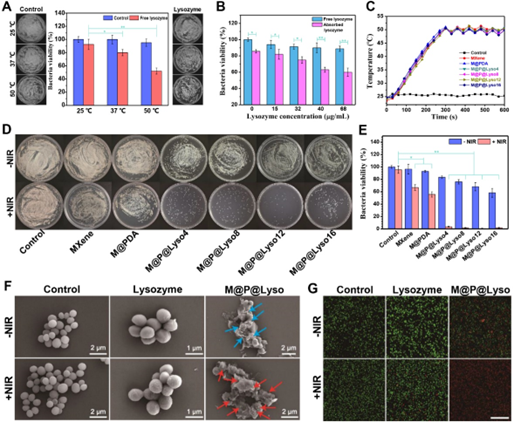
Figure 4. In vitro antibacterial activity of synthetic nanomaterials. A) Bacteriostatic rate of lysozyme at different temperatures (n = 3). B) Bacterial survival rates of free and immobilized lysozyme (i.e., M@P@Lyso) after 10 min of no light treatment (n = 3). C) The temperature curve of the bacterial suspension incubated with MRSA and different nanomaterials (50 μg/mL) under 808 nm laser irradiation. D) Photograph of bacterial colonies on LB agar plates. E) Bacterial survival of MRSA after different treatments. PBS served as a control group. F) Representative SEM images of MRSA with different treatments. G) Fluorescence images of cell live/dead assays of MRSA after different treatments. Scale bar: 50 μm.
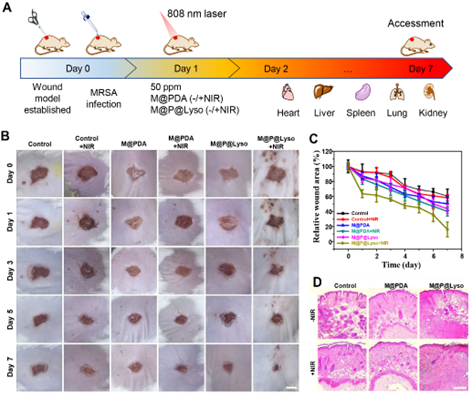
Figure 5. In vivo antibacterial performance study of M@P@Lyso+NIR. A) Schematic diagram of the establishment of the mouse wound model. B) Wound photos of mice treated with different treatments, with PBS-treated group as the control group, scale bar: 3 mm. C) Relative wound area of mice under different treatments. D) Histological images of H&E staining of mouse wound tissue after different treatments. Scale bar: 1.25 mm.
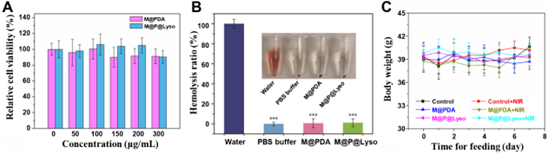
Figure 6. In vitro and in vivo biosafety analysis. A) Relative cell viability after treatment of 3T3 cells with different concentrations (0, 50, 100, 150, 200 and 300 μg/mL) of M@PDA and M@P@Lyso. B) Hemolysis rate and optical images of M@PDA and M@P@Lyso. C) Body weight change curve of mice in different treatment groups.
Summarize
In this paper, a composite photothermal nanoplatform composed of MXene and PDA was used to immobilize lysozyme and enhance the activity of lysozyme through the photothermal effect of MXene and PDA, thereby achieving efficient sterilization. In the synthesized M@P@Lyso nanocomposites, the excellent photothermal conversion ability of M@PDA provides the possibility for remote regulation of lysozyme activity. More importantly, the in vitro and in vivo antibacterial results show that M@P@Lyso+NIR can achieve about 98% bactericidal rate against MRSA, promote wound healing in mice, and have good biocompatibility. In conclusion, this work proposes an approach to address the threat of bacterial drug resistance through a stimulus-responsive enzyme nanoplatform, providing a new idea for the application of MXenes in the antibacterial field.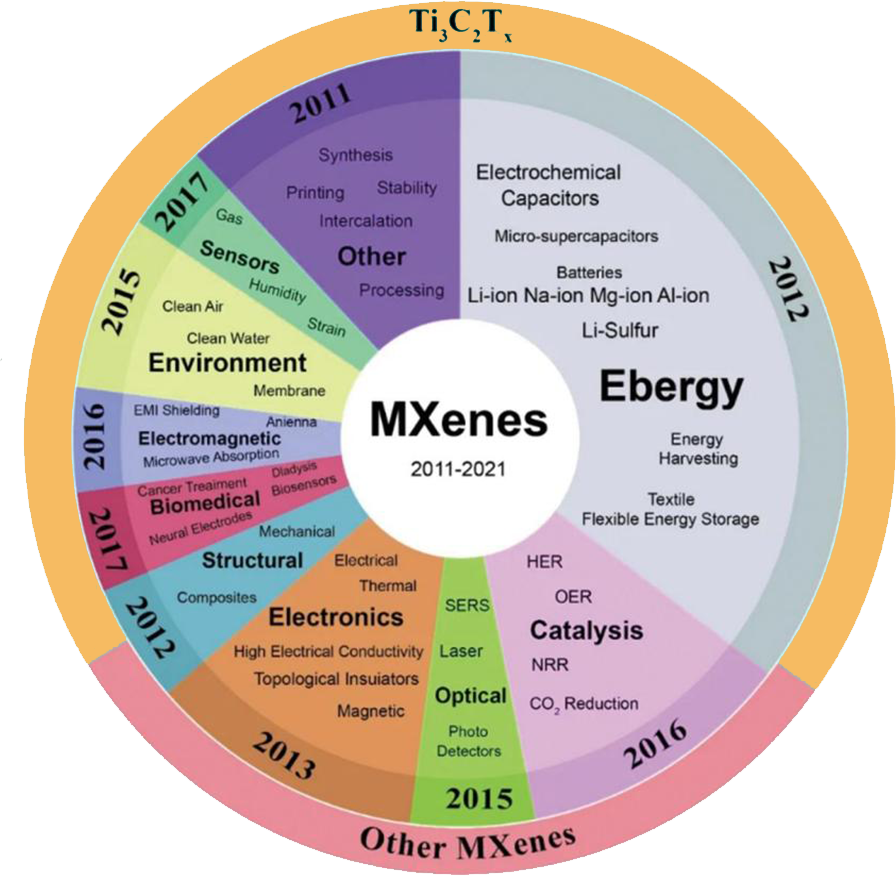
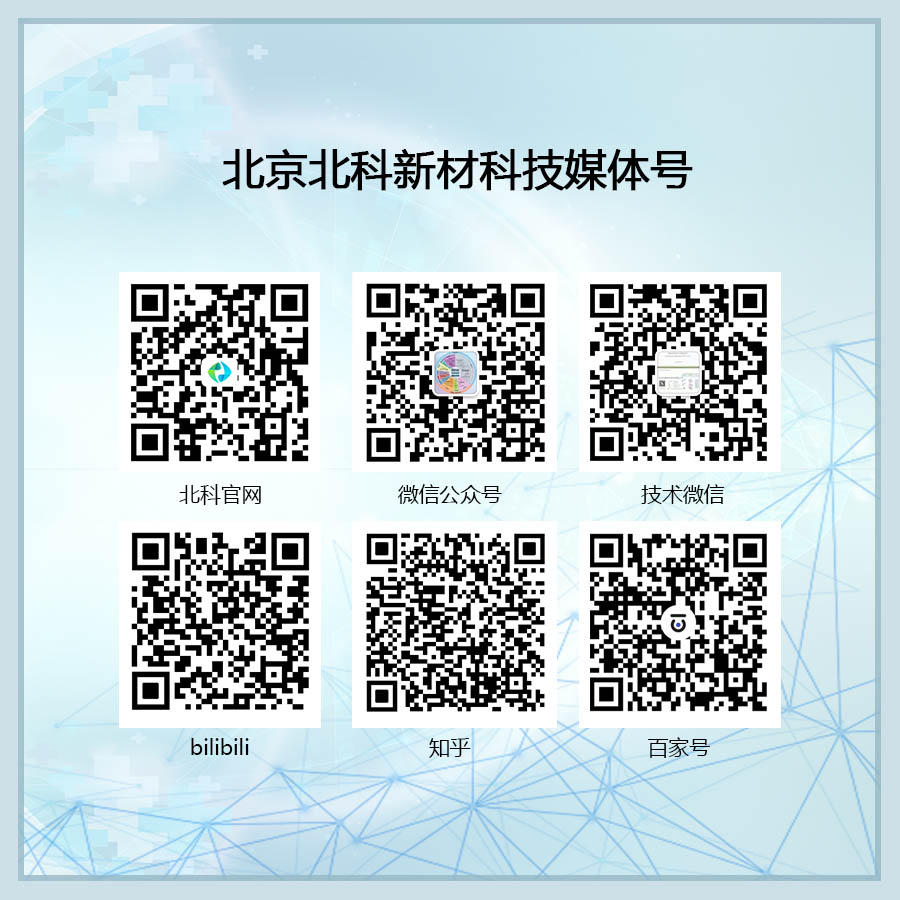
- Previous: Nano Energy|Molecular
- Next: MXene breakthrough: Na